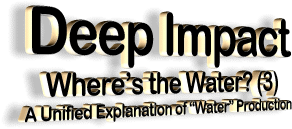
by Wallace Thornhill
Feb 17, 2006
from
Thunderbolts Website
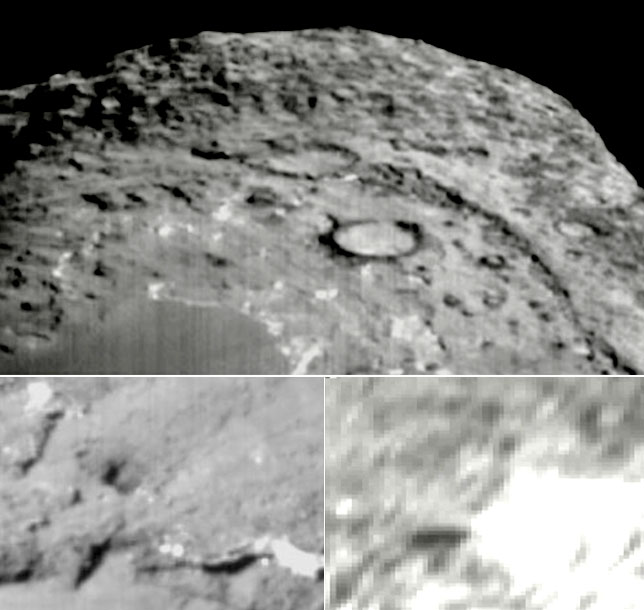
Credit:
NASA/JPL-Caltech/UMD
These close-up images of Comet Tempel 1, taken by the camera on the
impactor that
struck the comet nucleus, reveal white patches that have continued
to puzzle NASA scientists.
The idea of an electric comet traces to
scientific discussion in the second half of the nineteenth century.
In 1871, professor W. Stanley Jevons, suggested (in the journal
Nature) that comets might owe their “peculiar phenomena to electric
action”.
The following year Scientific American reported on the
research of,
“Professor Zollner of Leipsic”, who suggested that comet
tails, “which consist of very small particles, yield to the action
of the free electricity of the sun”.
Ten years later the electric comet had gained momentum. The 1882
English Mechanic and World of Science reported a,
“rapidly growing
feeling amongst physicists that both the self-light of comets and
the phenomena of their tails belong to the order of electrical
phenomena”.
By 1896, Nature could report:
“It has long been imagined
that the phenomenon of comet’s tails are in some way due to a solar
electrical repulsion”.
In retrospect it is clear that those envisioning electric activity
of comets were limited by traditional concepts of electrostatics,
concepts that have continued to breed misunderstanding into the 21st
century. But experimental knowledge of the “plasma universe” began
with Kristian Birkeland very early in the 20th century, not long
before Irving Langmuir named “plasma” for its life-like qualities.
Later, the groundbreaking work of Hannes Alfvén showed conclusively
that simple electrostatic formulae were wholly inadequate to account
for plasma behavior.
These things were unknown to the scientific community when Hugo Benioff published “The Present State of the Electric Theory of Comet
Forms” in 1920. Benioff acknowledged that,
“the outward radial
motions in all directions of particles close to the nucleus are best
explained as resulting from an electrical charge associated with the
nucleus”.
But as for the “repulsion” of comet tails, he said, this
required a charge separation beyond anything that could be
practically envisioned. So, to explain the behavior of comet tails,
he settled on the principle of “radiation pressure”, an idea to
which all of astronomy moved in the following decades.
Today, however, we know that comet tails can be influenced by solar
radiation “pressure”, but they are clearly not governed by it.
Nevertheless, the electric comet faded quickly as astronomers came
to envision an electrically inert, gravitationally dominated
universe. Earlier investigators, despite a comparative lack of data,
were more interdisciplinary. They could see certain features of
comets calling for an electrical explanation. But as specialization
took over, astronomers soon lost all interest in electricity, a
subject eventually banished from the training of astronomers and
disappearing completely from their vocabulary.
Was the progressive dismissal of electricity based on evidence, or
on something else? The comet provides a good illustration of the
point we’ve made many times in these pages. Theoretical assumptions
can marginalize uncomfortable facts to such an extent that they are
no longer noticed or remembered.
When 19th century astronomers wondered about the role of electricity
in comet behavior, they could see that a cometary coma, the
spherical envelope around the nucleus, could not be maintained by
gravity. But well before the full flowering of modern plasma
research, experiments showed that when a charged probe was placed in
plasma, a sphere of oppositely charged particles would gather around
the probe. The early researchers were only following the
experimental evidence when they recognized electrical phenomena in
the sphere of the coma.
Today our view of the comet is greatly enhanced by the technological
achievements of the twentieth century; but critical thinking—the
ability to question theoretical assumptions—has collapsed to the
point that astronomers barely notice the incongruities in coma
behavior.
Given the trivial gravity of a typical comet nucleus, the escape
velocity will be something like walking speed. Take a hop and you
will never return. Our visits to comets have shown material escaping
from the surface of nuclei in jets, some at supersonic speeds. The
jets throw material into space in all directions, at different
speeds and in irregular patterns. Then what happens?
A force that
you cannot find in the lexicon of astronomers gathers the material
into a spherical form, despite the fact that much of this material
is millions of kilometers or more from the nucleus and could not
possibly “see” the nucleus gravitationally. Nevertheless, in the
vacuum of space, as the comet speeds around the Sun, the nucleus
continues to hold in place the giant spherical cloud, up to 10
million kilometers or more in diameter.
As astronomers continued to evolve their gravitational models of the
heavens, the pioneers of plasma science explored the role of the
electric force, which is known to be 39 orders of magnitude
(1000,000,000,000,000,000,000,000,000,000,000,000,000 times) more
powerful than gravity.
Their explorations took them far beyond
electrostatics, to demonstrate the powerful dynamics of electric
currents in plasma and in high-energy plasma discharge. They
enumerated the attributes of “double layers” that gather as
spherical shells around charged objects in plasma. Across the walls
of such layers, they observed intense electric fields, while across
the larger distances between such layers the field could be much
weaker, even imperceptibly weak.
Plasma events are scalable. What occurs in the plasma laboratory can
occur on a vastly larger scale in space plasma. Hence, observations
of plasma behavior in the laboratory are a logical reference when
considering the mysteries of cometary comas—and that includes the
many enigmas that surround the identification of “water” in the
comas. According to the electric theorists, electricity can
accomplish the very things that have baffled the cometologists.
In their analysis of the coma, astronomers begin with the assumption
that water is evaporating in the heat of the Sun, off the surface
ices of the nucleus. They do not “see” the water, but call upon the
effects of solar radiation (photolysis) on assumed “water” to
account for the abundant hydroxyl radical OH (oxygen-hydrogen
molecules) in the coma.
In our previous Picture of the Day we noted another possibility.
Astronomers have not considered the energetic ionic chemical
reactions that would accompany plasma discharge “sputtering” of a
cathode or negatively charged object in space. Production of OH
would be virtually certain if proton streams sputtered material from
the surface in the fashion that the electric theorists have claimed.
When theoretical issues arise, the contrast between predicted
behavior under the two vantage points becomes a distinct advantage.
With this advantage in mind we offer the following summary of facts
and contrasting interpretations.
-
Negatively charged nucleus.
The electric view compares the
behavior of negatively charged probes in plasma experiments to the
behavior of comets. It therefore predicts a spacecraft moving
through the coma would encounter a number of plasma sheaths or
double layers as it approached the nucleus of a comet. Plasma
sheaths will form between regions in which the characteristics of
the plasma itself change.
Across a sheath the voltage differential
between the comet nucleus and the solar wind should show up most
dramatically. Positive ions should "pile up" on the sunward side of
the sheath in the coma’s electrical response to the solar wind. In
fact, this was observed at both comets Hyakutake and Hale-Bopp and
surprised researchers by its unexpected stability over "hours, days
and even weeks."
The researchers were surprised because they had imagined that the
concentration of ions was a mechanical “bow shock” as material in
the jets encountered the solar wind. Since the jets are highly
variable, the intensity of the “bow shock” should vary accordingly.
However, plasma sheaths respond only to the electrical environment,
which will be less variable than episodic jets, and will be most
concentrated in the sunward direction, precisely as observed.
Neutral oxygen (O) near the nucleus shows a spectral line indicative
of the presence of an "intense" electric field. So the electric
model anticipates energetic "hot" electrons and negatively charged
ions close to the nucleus, as sputtering strips atoms and molecules
directly from negatively charged rock. The International Cometary
Explorer (ICE) mission to comet Giacobini-Zinner found "hot
electrons coming back more and more frequently." The Halley probes
detected “very energetic electron populations” in the coma. And the
presence of negatively charged ions surprised the investigators.
They wrote,
In fact, the intense electric field near the comet nucleus makes no
sense whatsoever if a comet is merely an inert body plowing through
the solar wind. Electric currents produce magnetic fields, and
"magnetized cometary plasma … is much larger than was theoretically
predicted" [emphasis ours], according to the 1986 Nature report on
Comet Halley.
-
OH production.
If one accepts the evidence that a comet is a
negatively charged body moving through the weak radial electric
field of a positively charged Sun, the production of OH in the coma
will not look anything like the standard picture.
Taken as a whole, the facts we have already summarized (here and
here), virtually preclude abundant water on the comet nucleus, while
the sputtering hypothesis stands out in its consistency with all
available data. In the electric model, negative oxygen ions will be
accelerated away from the comet in energetic jets, then combine
preferentially with protons from the solar wind to form the observed
OH radical and the neutral hydrogen gathered around the coma in vast
concentric bubbles. The reactions simply confirm the energetic
charge exchange between the nucleus and Sun.
It is interesting to note that the warning signs for standard theory
came very early. Even before the first visit to a comet, a 1980
report in the journal Nature outlined some of the mysteries and
anomalies.
It concluded:
This cautionary note was not heeded. Later, in 1986,
Nature reported that OH issues remained perplexing and "may indicate
the existence of parents of OH other than H2O”. But in the years
that followed, despite the shocking failure of the “dirty snowball”
model to predict any milestone discoveries, one of the most critical
questions simply disappeared from scientific discussion.
-
Too much atomic hydrogen.
Early in the 1970s, astronomers were
stunned when they observed cometary comas in ultraviolet light. They
discovered immense envelopes of fluorescing hydrogen atoms much
larger than the visible coma. In the case of Comet Bennett the
hydrogen coma was an "almost unbelievable" 15 million kilometers in
diameter.
That's 10 times the diameter of the Sun! Where did this
immense volume of atomic hydrogen come from? The prevailing theory
of OH production requires some sort of balance between OH and
neutral hydrogen. Whatever the difficulties faced by the standard
model explanation of the spherical coma, the difficulties can only
grow in relation to a hydrogen envelope millions of kilometers in
diameter.
-
Plasma sheaths and “double layers”.
Many features of the electric
model of the comet derive from the laboratory behavior of
electrified plasma and plasma discharge. In an electrically neutral
environment nothing comparable to the sheaths that occur around
charged bodies in plasma will be expected. Comet researchers working
with the “dirty snowball” model of a comet expected no such
phenomena.
Across the wall or boundary of a plasma sheath—what plasma experts
call “a double layer”—an intense electric field may occur in
contrast to a weaker field between these boundaries. Variations in
the energies of charged particles will contrast sharply with what
would be expected in an electrically neutral environment.
This is exactly what occurred as Giotto and the two Vega spacecraft
moved through Halley’s coma. The Nature reports are replete with
references to unexpected variations in charged particle energy
levels—
The report notes three regions of variation in ion (charged
particle) characteristics. An outer region “contains pick-up ions in
the solar wind". This may be interpreted electrically as the outer
edge of the comet's plasma sheath. A second region inside the
so-called “bow shock” stretches for several thousand kilometers,
revealing the most intense fluxes and distinct intensity spikes.
This may be the crossing of the double layer, where a strong radial
electric field exists. A third region is characterized by lower
intensities, but with sharp spikes at closest approach. Here we may
be seeing the cometary plasma being disturbed by the accelerated
ions and electrons from the comet jets.
In Vega 1’s close approach, narrow peaks were evident “at all
energies”. The report says,
Of course, the magnetic field measures
the strength of the electric currents flowing near the comet.
Finally, at closest approach, there was a sudden increase in highly
energetic electrons. “No significant variation in this flux had been
observed for several days preceding closest approach”.
At a distance of 40,000 kilometers from the nucleus, the Vega 2
craft detected a surge in cometary plasma density,
-
“accompanied by
large fluxes of suprathermal electrons with energies up to a few keV”
[emphasis ours].
-
“The most dramatic effects were observed in the last minute before
closest approach… two short bursts of ions with energies up to 400
eV were observed: During the last 45 sec before closest approach,
the flux increases rapidly until the spacecraft appears to be
surrounded by a dense and very hot cloud of plasma… the energies are
very much higher than had been anticipated”.
For these “energies of
the observed ions” the researchers had no explanation.
-
X-rays.
In1996, the German X-ray Roentgen Satellite (ROSAT)
viewed the comet Hyakutake. The astronomers hoped to see a small
smudge at best and some wondered why anyone would bother. X-rays had
never been detected from a comet before and theorists could only
imagine a few ways that a comet could produce any x-rays at all. So
the astronomers were shocked to find x-rays up to 100 times more
intense than even the most optimistic predictions!
Also the emission
flickered on a time scale of hours.
-
"We were prepared to see
nothing. So it was an enormous surprise when this thing was just a
boomer," said a team member. A NASA report noted, "…there must be
previously unsuspected 'high-energy' processes taking place in the
comet…"
This was the last thing that the standard model would have
anticipated, but an electric current in a near vacuum is the way we
produce x-rays on earth. The flickering is characteristic of a glow
discharge. An intense electric field in a cometary double layer can
accelerate electrons and cometary ions so that they collide with
solar wind ions and emit x-rays.
It is significant that the x-rays
did not come from a region expected by a “mechanical shock”
model—the only model available to the surprised astronomers. They
came from a crescent-shaped region in the direction of the Sun,
which is where we should expect the maximum electrical stress.
Following this chance discovery, researchers have become accustomed
to x-rays from comets, but the uncompromising implication of an
electrical transaction, or charge exchange, between the comet and
the Sun has yet to sink in.
-
Flare-ups in deep freeze.
In 1991, comet Halley flared up to 300
times its normal brightness between the orbits of Saturn and Uranus,
14 times further than the Earth from the Sun. The comet's surface
should be at -200 ˚C and "no kind of chemistry can work that far out
from the Sun." No theory could explain the outburst from the
15-kilometer nucleus, which created a cloud of dust 300,000
kilometers wide. The cloud was "made mainly of dust, with no sign of
any spectral lines emitted by any gas."
Significantly for the
electrical model (which does not require any gas from heated ices to
explain the outburst) the Sun was going through a maximum of
activity that fitted the outburst of comet Halley.
Astronomers could
not see the significance:
But comments such as this require one to
exclude electrical currents from consideration.
A high voltage,
negatively charged comet will attract protons to the nucleus from a
huge volume of surrounding space.
-
Surface erosion.
In the electric model as formulated by Wallace Thornhill, “cathode sputtering” will disintegrate surface layers of
the negatively charged object by bombarding it with energetic ions
in an electric discharge. The discharge will be concentrated in
small spots as arcs eat away a surface, giving rise to steep-walled
craters and broad flat-floored valleys surrounded by sharply-defined
mesas or terraces.
That is the familiar look of electrical etching.
A beautiful example is seen on the surface of Comet Tempel 1 above,
but other examples are abundant on planets and moons. The
electrically etched surface of Jupiter’s moon Io is the most
striking example because the process is still underway in the
electrified Jovian environment.
Significantly, a paper in the journal Science, in October 2005,
noted that “shocks” caused by ion sputtering of a cathode or
negatively charged surface sharpen steep surface features—a dramatic
contrast to the way the evaporation of ices will attenuate surface
relief. A steep “cliff” remains even as it is eaten away by an arc
progressively expanding the dimensions of the valley floor.
As Thornhill has long contended, cathode arcs tend to impinge on
sharp edges because of the higher electric field there—a point that
reinforces the Science article. In contrast to prevailing ideas
about Io’s “volcanoes”, Thornhill predicted that the
electric-discharge plumes would move around the edges of the valley
floors. And that is what the Galileo probe discovered—another
surprise for astronomers and planetary scientists who had not
expected to find “volcanoes” to be moving across the surface of Io.
Viewing the comet in similar electrical terms will allow us to
answer one of the least noticed but most profound mysteries posed by
Comet Tempel 1. In the best pictures of the nucleus taken from the
spacecraft, numerous patches of whiteout appear, most frequently on
the edges of mesa cliffs, crater walls, and other surface relief. It
is clearly not a random glitch in photography. Somehow the camera,
photographing a body as dark as copier toner, was selectively
saturated by bright spots on the surface.
Have we ever seen such a thing before? Curiously, exactly the same
thing occurred when the Galileo probe viewed the electric plumes
moving across the surface of Io. The designers of the camera had not
anticipated anything so bright. But that is the nature of the
electric arc—it’s why arc welders wear those darkened masks!
What will it take, then, to convince NASA scientists to ask the
question: Do the patches of whiteout in close-ups of Tempel 1 reveal
electrical discharge activity—on a scale that would immediately
invalidate the foundational assumptions of today’s cometary science?
-
Fine cometary dust.
Cathode sputtering has an effect that is
simply “beyond the reach” of evaporating volatiles. It can create an
exceedingly fine dust down to 1 micrometer or even finer. (One
micrometer is just 40 millionths of an inch). This unique capability
of cathode sputtering is why the process is used in the manufacture
of highly reflective mirrors for modern telescopes. So again, a
comparison of practical electrical technology with the discoveries
of Deep Impact is only reasonable.
This line of investigation introduces another surprise: Astronomers
could not understand what occurred when the 800-pound projectile hit
the comet nucleus. An enormous volume of an extraordinarily fine
dust was thrown into space at high speed, creating an extremely
bright cloud due to the dust’s remarkable reflectivity. NASA
scientists estimated that the dust particles were only .5 to 1
micrometer in diameter.
But was the surprise justified? Almost twenty years earlier the
visit to Halley had investigators wondering how “sublimating ices”
could produce such fine comet dust. But that surprise, like so many
others, seems to have been quickly forgotten.
Also from the report in Science, in its recent report on the Deep
Impact explosion:
Remembering that the water was estimated from OH molecules seen
after the impact, we can see that another key prediction by
Thornhill, made in October 2001 concerning the expected outcomes of
the impact with Tempel 1, was satisfied:
In
the electric view, the unexpected energies of the Deep Impact
explosion, and the release of unexpectedly fine dust, are both the
predictable consequence of plasma discharge.
|