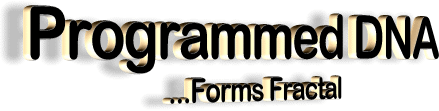
by Kimberly Patch
TECHNOLOGY RESEARCH NEWS
March 28, 2005
from
AllBusiness Website
Winfree's research
colleagues were Paul W. K. Rothemund and Nick
Papadakis.
The work appeared in the
December, 2004 issue of Plos Biology. The research was
funded by the National Science Foundation (NSF),
the Defense Advanced Research Projects Agency (DARPA),
the National Aeronautics and Space Administration (NASA),
and GenTel.
Please direct queries about this article to TRN at
editor@trnmag.com (c)
Copyright Technology Research News, LLC 2000-2005. All
rights reserved. -
http://www.trnmag.com/
(Distributed via M2 Communications Ltd -
http://www.m2.com) |
A decade after the idea became the topic
of his doctoral dissertation, a researcher at the California
Institute of Technology has showed that it is possible to coax short
strands of artificial DNA to spontaneously assemble into a
Sierpinski triangle.
A Sierpinski triangle is a type of crystal, or structure that
regularly repeats. The Sierpinski triangle is
fractal - a pattern of triangles
that looks the same in zoomed-in or zoomed-out views. The ability is
a step toward embedding programming instructions in chemical
processes.
This is a corollary to the way computer
instructions are embedded in everything from automobile engines to
cell phones via microprocessors.
"Programmable embedded control makes
things possible that were virtually inconceivable," said Erik
Winfree, an assistant professor of computer science at The
California Institute of Technology.
The DNA Sierpinski triangles show
that there is no theoretical barrier to using molecular
self-assembly to carry out any kind of computing and nanoscale
fabrication, according to Winfree.
If someone comes up with the right
rules, the right set of molecules should be able to carry out the
instructions, he said.
This type of algorithmic self-assembly is a testing ground for
learning how to embed logical rules within a molecular system so
that information processed by the molecules themselves is
responsible for directing the local processes, said Winfree. In the
case of a Sierpinski pattern, the molecules are directing the
process of self-assembly, he said.
Although today's technology does not have electronics-like control
of chemical and molecular-scale processes, biology does.
"The only place one finds
sophisticated embedded control of chemical processes is in
biology, where biochemical information processing controls,
orchestrates [and] organizes all of life's functions."
Algorithmic self-assembly can be thought
of as an extremely simplified version of organismal development,
said Winfree.
Coaxing artificial strands of DNA to
form a Sierpinski pattern is,
"a far cry from an organism," he
said. "But it is also far more complex than the four DNA rule
tiles that directed its growth."
DNA is made up of four bases - adenine,
cytosine, guanine and thymine - strung along a sugar-phosphate
backbone.
Adenine and thymine, and
cytosine and guanine can combine with each other.
Biological DNA forms the familiar double helix when a pair of single
strands that contain matching bases combine and coil up. Researchers
can make artificial strands form DNA tiles by engineering stretches
of one strand that match another strand.
The researchers formed short strands of DNA capable of combining
into tiles that represent logic rules, short strands capable of
combining into tiles that represent input, and long nucleating
strands.
They mixed the strands, heated the
solution, then let it cool slowly over several hours.
"At about 60 to 70 degrees Celsius,
the tiles spontaneously self-assemble from their components
strands, but it remains too hot for the tiles to associate with
each other," said Winfree.
At the same or a slightly lower
temperature, the input tiles stick to the long nucleating strands.
And somewhere between 30 and 40 degrees
Celsius the rule tiles begin to assemble onto the nucleating
structure to form, tile by tile, and layer by layer, the algorithmic
crystal.
"In some, few errors occur, and the
Sierpinski pattern emerges intact."
The researchers have made Sierpinski
patterns on surfaces and more complicated Sierpinski triangles
in solution. Sierpinski triangles involve more types of
tiles. Some of the researchers' triangles were as large as one
micron, or thousandth of a millimeter.
The keys to the researchers' success was using the long nucleating
DNA strands to get things started and a better microscope technique
to see what was happening. The errors were as interesting as the
successful Sierpinski patterns.
The experiments' error rates ranged from
1 to 10 percent.
"We expected lots of errors, but we
didn't expect the kinds of errors that we saw," he said.
In general, several errors would
normally increase the randomness of a pattern.
However, there were places within some
samples where several errors conspired to create large patches of
zero tiles or to perfectly terminate nascent Sierpinski triangles at
the corners, said Winfree.
"Such coincidences should be so rare
that one would never see a single instance in one million
crystals," he said.
The researchers have a hypothesis
capable of explaining how these correlated errors arise,
"but it remains to be proven," said
Winfree.
The researchers were also surprised to
see that one of the tile designs, instead of simply forming
two-dimensional sheets, formed a long tube with the sheets rolled
up. The DNA nanotubes are similar to but 10 times larger than
carbon nanotubes, which are
rolled-up sheets of carbon atoms that form naturally in soot; they
are more similar to protein microtubules that self-assemble as part
of the cellular cytoskeleton.
Carbon nanotubes can be narrower than a single nanometer, or 5,000
times narrower than a red blood cell. A nanometer is one millionth
of a millimeter.
The researchers are working to decrease the method's error rate.
"We have developed some ideas for
how to embed error correction within the crystal growth process
- somewhat analogous to error-correcting codes in information
theory - and we are now trying to experimentally demonstrate
this scheme," said Winfree.
If they are successful in reducing
assembly errors to insignificant levels,
"as the theory optimistically
predicts creating complex structures by self-assembly becomes a
form of programming," said Winfree. "If you can conceive of a
logical method for growing your structure, then it will work in
practice," he said.
|