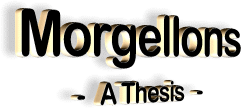
by Clifford E. Carnicom
Carnicom Institute
October 15, 2011
from
CarnicomInstitute Website
Note:
I am not offering any medical advice or
diagnosis with the presentation of this
information.
I am acting solely as an
independent researcher providing the results
of extended observation and analysis of
unusual biological conditions that are
evident.
Each individual
must work with their own health professional
to establish any appropriate course of
action and any health related comments in
this paper are solely for informational
purposes and they are from my own
perspective.
Abstract
A substantial body of research has accumulated to make the case that
the underlying organism (i.e., pathogen) of the so-called "Morgellons"
condition, as identified by this researcher, is using the iron from
human blood for its own growth and existence.
It will also be shown that the
bio-chemical state of the blood is being altered in the process. The
implications of this thesis are severe as this alteration affects,
amongst other things, the ability and capacity of the blood to bind
to oxygen. Respiration is the source of energy for the body.
This change is also anticipated to increase the number of free
radicals and to increase acidity in the body. This process also
requires and consumes energy from the body to take place; this
energy supports the growth and proliferation of the organism. The
changes in the blood are anticipated to increase its combination
with respiratory inhibitors and toxins.
The changes under evaluation may occur
without any obvious outward symptoms.
It is also anticipated that there are
consequences upon metabolism and health that extend beyond the
functions of the blood. This change represents essentially a
systemic attack upon the body, and the difficulties of extinction of
the organism are apparent. Physiological conditions that are in
probable conjunction with the condition are identified.
Strategies that may be beneficial in
mitigating the severity of the condition are enumerated.
This paper will present this case progressively, and it will build
upon the information that has been presented in previous papers.
As we continue with our discussion, there will be three different
general approaches that will be used in a combined sense to reach
the conclusions that have been stated above.
-
the first of these will be
direct observation
-
the second will be qualitative
chemical examination
-
the last will be the use of
spectral analysis and analytics
A synthesis of each approach will give
us the understanding of the situation that we require.
Let us begin with some discussion on the
chemistry of iron and then follow with a few of the qualitative iron
tests that are helpful in the methods that have been developed.
1. A Brief
Introduction to the Chemistry of Iron
Let us start with an introduction to
iron.
Iron exists in three primary forms in
nature:
the first in its elemental form with no net charge, and the
other two as compounds, known as ferrous and ferric compounds.
It is
these latter two states of iron that will be of interest to us in
terms of human biochemistry.
Ferrous compounds involve iron in a charged state, known as Fe2+,
and ferric compounds involve iron in the valence state of 3, or
Fe3+. The term
valence refers to the number of electrons lost or
gained in a chemical reaction. For example, a loss of two electrons
from an atom will leave the atom in a charged state of +2.
A charged
atom or compound is called an ion or ionic compound, respectively.
Why is this important to us and why should we learn about the
chemistry of iron? It is because iron is in our bodies and it is
absolutely crucial to our lives and our health. The charged state of
the iron in our bodies and our blood is of the utmost importance in
understanding the changes to human health that are occurring.
Now let us start focusing upon the iron in blood. Your blood needs
iron to function. Not only does your blood need iron to function but
it needs the iron to be in a particular state for your blood to work
properly.
The iron in your blood must be in the
ferrous form, or the Fe2+ in order to bind to oxygen.1,2,3,4,5
If it is not in this state (e.g., ferric
iron or Fe3+), it will not bind to oxygen and human health will
suffer. You are not thriving in an energetic sense if you do not
have the proper oxygen content within your blood.
Hopefully we understand that the state of the iron in our bodies is
not a trivial affair and it is in our interest to become educated on
the matter. It is the very path that I have chosen in this research
and the implications of these studies are profound.
Now let us talk, in a general sense, about what causes iron to
change state. What for example, would cause iron in the elemental
form (Fe) to go to the Fe2+ (charged) state, or for that matter,
from the Fe2+ state to the Fe3+ (further charged) state?
It is here that we introduce and explain
the term of oxidation.
As a familiar example, when something
rusts, it is being oxidized. What it means, in a more descriptive
sense, is that a chemical reaction is taking place and that
electrons are being removed from an atom or substance. Formally
speaking, oxidation refers to the process of losing electrons.
Oxidation increases the charge state of
the atom or ion, because as an electron (i.e., negative charge) is
removed, the atom, ion or substance becomes more positive as a
result. A typical example of oxidation is the change of iron from
the Fe2+ state (i.e., ferrous) to the Fe3+ (i.e., ferric) state
mentioned above.
Below are some photographs that show testing of the iron ion in
varying oxidation states, i.e., Fe2+ and Fe3+ with the use of some
specialized chemical reagents.
One of the factors that is important in
the qualitative tests that we are doing is that of color; color is
an extremely useful tool for determining the existence of metals in
solution and for the chemical state that they are in.
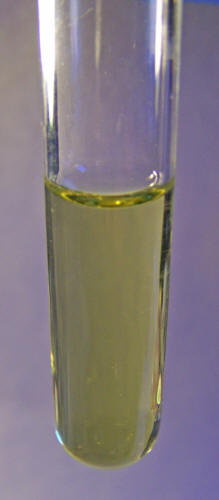 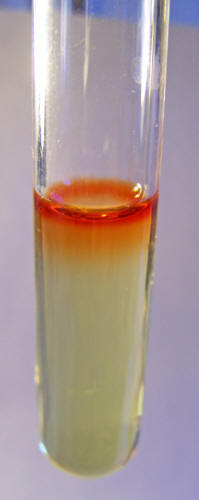
This set of photographs shows a solution of what is called "liquid
iron", essentially a solution of a ferrous salt (with some minor
impurities) that is used in gardening applications.
This ferrous solution is formed from a
representative iron salt with the iron in the Fe2+ oxidation state.
One of the important characteristics visually of the Fe2+ iron is
the greenish tint that often accompanies the Fe2+ iron oxidation
state.
The photograph to the right shows the
addition of a chemical (1,10 phenanthroline) that is very sensitive
to the presence of the Fe2+ ion, and it turns the solution red in
combination with the ion.
The use of this chemical is a valuable
and sensitive qualitative method to determine the existence of the
Fe2+ ion.
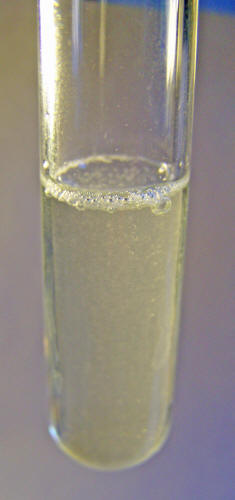 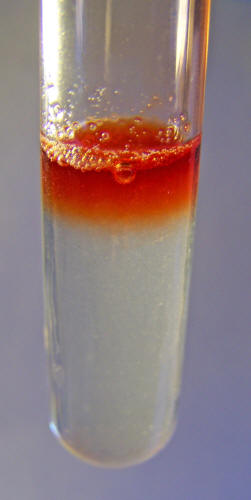
This set of photographs is provided to demonstrate the variability
of color as well as its value and importance.
The photographs above show a freshly
dissolved solution of ferrous sulfate. When the ferrous sulfate is
dissolved in water it will ionize (separate into ions of Fe2+ and
(SO4)2-).
It will also generally turn light green
in color but this example lacks the stronger green tint shown in the
set to the left. Colors can easily be influenced by concentrations
and impurities. A separate solution made previously demonstrates a
stronger green tint that is characteristic of the Fe2+ ion; this
particular one does not.
The use of 1,10
phenanthroline reagent
resolves the issue very clearly, however, as the characteristic
reaction to produce the bold red color in combination with the Fe2+
ion is evident.
This example demonstrates the value of
approaching the problem from more than one perspective, such as with
the use of color, chemistry and spectral analysis for a more
comprehensive assessment of the situation.
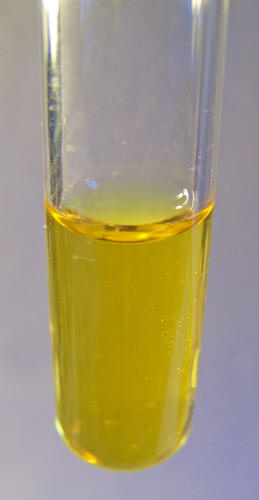
This set shows an analogous qualitative chemical test for the
presence of the Fe3+ ion solution.
This particular solution is that of
ferric chloride. There is an expected similarity in color between
various ferric salts, as the ionic form of iron is the agent
responsible for the color.
A distinctive feature of the Fe3+ ion in
solution is that of a yellow to brown color.
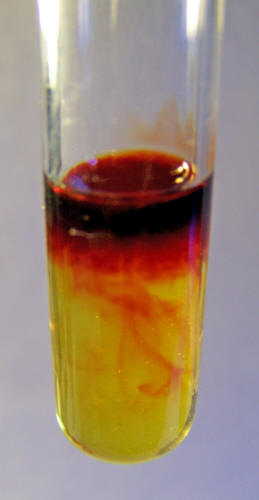
This photo also shows the use of a different, but equally important,
reagent that is used to detect the presence of the Fe3+ ion in
solution.
The chemical used in this case is that
of sodium thiocyanide. Even though this reagent also produces a bold
red color, this test and the one mentioned above using 1,10
phenanthroline are entirely separate and unique from one another,
and are only valid for the particular ion of each test.
The value of the tests shown above are threefold:
-
First we have a sensitive
qualitative method of identifying the existence of specific
iron ions, i.e., Fe2+ and Fe3+ in solution6. These tests can
also be extended in combination with a spectrophotometer to
provide concentration levels of the ions, if required.7
-
If the test succeeds, we know
that the iron states are present in an ionic form within the
solution. If the test fails, it does not mean that Fe2+ or
Fe3+ are not present, it only means that they are not
present in ionic (i.e., disassociated) form. It is possible
that the iron could exist in a different form (e.g., bound
within a molecular compound) than ionic, and the test would
not show this fact. This distinction will become important
in later testing procedures that are described.
-
Regardless of individual
variations, there is a clear and distinctive difference
between the greenish tints associated with the Fe2+ ion and
the yellowish and brown tints that result from the Fe3+ ion.
This distinction will also become important in later
testing.
2. Beginning
Observations
Let us now switch over to the course of direct observation.
Many of us may recall that certain
culture growth trials were discussed in an earlier paper entitled "Morgellons
- A Discovery and a Proposal." 8
In that paper, conditions and
circumstances that both increased and inhibited the rate of growth
of the organism were discussed. A section of that paper again is
relevant again with direct observation, as shown below, in
combination with the color characteristics of iron discussed above.
Direct observation essentially indicates to us that the organism is
able to utilize and absorb iron in the Fe3+ state.
Let us discuss further why this is the
case.
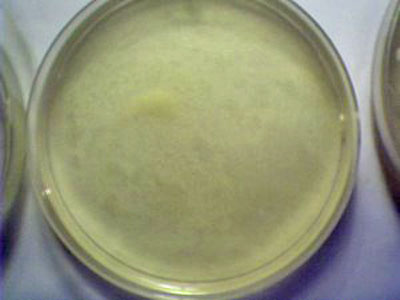
This photograph shows a culture that has just been started.
The process of starting a culture with
this method requires only a single drop of the culture solution. The
culture solution is prepared by subjecting the pulverized and dried
filaments of previous growth to sodium hydroxide in solution and
heat to the boiling point. The culture medium has ferrous (Fe2+)
sulfate and hydrogen peroxide added to it as described in the paper
referenced.
This chemical reaction that takes place
will again be described in more detail below.
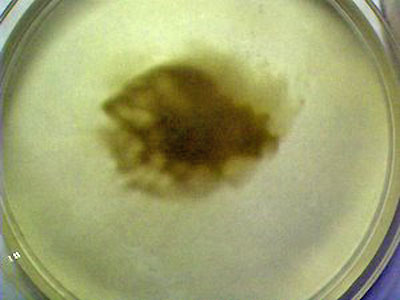
This photograph shows the state of the culture growth after a few
days have elapsed.
The dark brown color characteristic of
the ferric (Fe3+) oxidized iron within the organism growth is
visible. The organism is absorbing the nutrients that have been
provided in the culture medium. In this case, the Fe2+ ion
originally introduced into the solution was oxidized by the hydrogen
peroxide (Fenton's reaction) to produce the Fe3+ iron state.
The organism is able to nourish itself
from this oxidized state of iron and it imparts the characteristic
color of the iron (Fe3+) oxidation state within the growth of the
culture.
In order to understand the results of the photographs above, it is
helpful to describe a chemical reaction, called "Fenton's reaction"
that was discussed in the former referenced paper.9 Fenton's
reaction involves the combination of iron in the Fe2+ state (in this
case, ferrous sulfate) and hydrogen peroxide.
The reaction is as follows:10
Fe2+ + H2O2 --> Fe3+ + OH + OH−
This reaction was established in the
following manner:
A starter culture of the underlying
organism was introduced into distilled water. A few drops of a
ferrous salt solution, namely ferrous sulfate was introduced
into the culture.
This was followed by a few drops of
hydrogen peroxide. It has been learned that this culture medium
rapidly accelerates the growth of the culture.
The result of the combination of the
iron in the Fe2+ state with hydrogen peroxide produces three things:
-
Iron ions in the ferric state, or Fe3+
-
The hydroxide radical, OH-
-
The hydroxyl radical, a highly reactive free radical.
Notice that none of these three developments were dependent upon the
culture; Fentons reactions would have taken place regardless of
the introduction of the organism.
What we do know from the reaction,
however, is that the iron is oxidized to the Fe3+ state and becomes
immediately available to the organism along with the hydroxyl
radical. The paper mentioned discusses some of the ramifications of
this combination with respect to health.
Not only does the oxidation takes place,
but we see that the organism is directly able to utilize the iron in
this oxidized state (Fe3+) for its growth and sustenance.
This provides our first link in understanding the role of oxidation
of iron in our body and its relationship to the growth of the
organism.
All of the conditions described for the
controlled petri dish trial are also to be found to occur within the
human body.
3. Qualitative
Chemical Analysis
There are chemical tests which can be performed to determine the
existence of substances, particularly those in ionic form.
These tests are valuable in that they
are relatively simple and yet they can provide crucial information
as to the existence of a metallic ion, for instance, without
providing quantitative or concentration levels.
Examples of this
include the determination of the existence of the iron ions (both
ferrous and ferric), copper ions, sulphate ions, chloride ions and
others. 11,12,13
It is important to understand that the
tests being described in this section are for ionic forms only, i.e.,
they are in a disassociated form in solution. A negative test does
not mean that the element in some form does not exist, (.e.g, bound
in a molecular form); it only means that it does not exist in an
ionic form. This distinction will become important to us as we
proceed later with additional laboratory procedures.
An excellent example of a qualitative test for the presence of ionic
forms of iron has already been described in the earlier section of
this paper, entitled An Introduction to the Chemistry of Iron.
In
this case, as described, certain reagents were used to positively
identify the presence of the Fe2+ and Fe3+ ions in known solutions.
Now let us apply these methods to the questions at hand, which are
twofold:
-
Does human blood in solution contain iron ions? We know that
blood contains iron, so it will be of interest to examine if it
exists in ionic form.
-
Does the culture solution (as developed from oral filaments
characteristic of Morgellons) contain iron ions?
Let us discuss the first question, i.e., does blood contain iron in
ionic form? If so, is it in the Fe2+ state, or the Fe3+ state, both,
or none?
We can answer this question with the
application of the same reagents mentioned earlier, 1,10 phenanthroline for the test of Fe2+ ions and sodium thiocyanide for
the testing of Fe3+ ions.
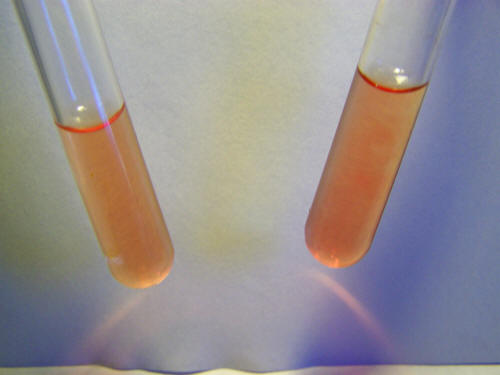
Testing for Fe2+ ions in blood in distilled water solution with1,10
phenanthroline. Results are negative. No characteristic deep red
color forms in the test tube to the right where the reagent has been
added.
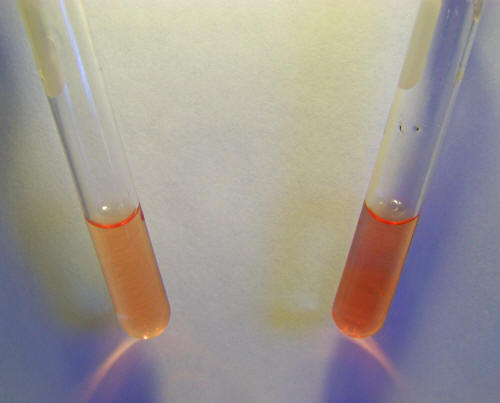
Testing for Fe3+ ions in blood in distilled water solution with
sodium thiocyanate. Results are negative. No characteristic deep red
color forms in the test tube to the right where the reagent has been
added.
The results in both cases are negative.
This means that human blood
does not show the existence if iron in ionic form, either Fe2+ or
Fe3+ within it. It does not mean that blood does not have iron
within it, for we know that it does.
But in what form does it exist
then? If it is not ionic, is the iron bound in some way? If so, what
is it bound to? How do we know what state it is in (Fe2+ or Fe3+) if
it is bound to something?
These are some of the questions before
us.
The answers to these questions will
become important to us in our understanding of any changes taking
place to the blood and they will become equally relevant in our
tests of the culture solution based upon oral filament growths. This
result also raises the problem of how do we go about qualitatively
testing for iron in the blood as we have now learned that the direct
ion testing approach is not sufficient.
As we proceed, please keep in the forefront that our problem is to
approach the question of how the state of oxidation of blood is
affected by the Morgellons condition.
Now let us test the culture solution in the same way: The
preparation of the culture solution can be described in detail at a
later time; this has been summarized to some degree in previous
papers.
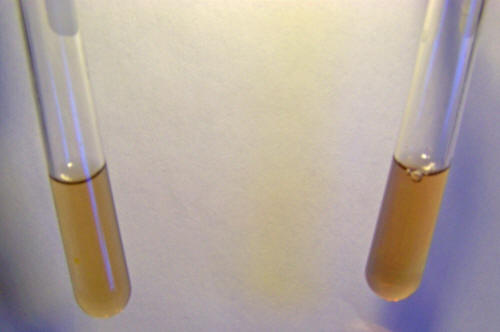
Testing for Fe2+ ions in the culture solution with1,10
phenanthroline. Results are negative. No characteristic deep red
color forms in the test tube to the right where the reagent has been
added.
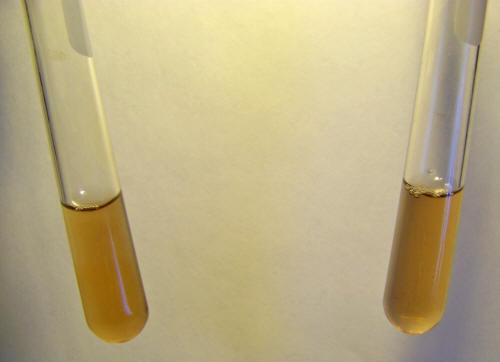
Testing for Fe3+ ions in the culture solution with sodium
thiocyanate. Results are negative. No characteristic
deep red color forms in the test tube to the right where the reagent
has been added.
The results are again in both cases negative.
This tells us
correspondingly, that the culture solution does not contain iron in
the ionic form (Fe2+ or Fe3+), at least to the degree of sensitivity
of the tests. Once again, it does NOT mean that the culture
solutions do not contain iron, only that if it is present that it is
not in the ionic (disassociated) form.
The issue, therefore, must provoke our
testing methods further and the question of iron binding to other
molecules, even if in an oxidized state (Fe2+ or Fe3+), rises to
importance.
4. An
Introduction to Bonding
Ionic, Covalent, Polar Covalent and Coordinated
Covalent Bonds
Soon we must educate ourselves further on how iron exists within the
blood.
Before that occasion, however, we must
also spend some time talking about the various methods that atoms
use to bind together to form molecules and compounds. Much of what
happens in chemistry is in some way related to bonding and it is
helpful to have at least some background on the subject. Ultimately,
the knowledge is crucial to our understanding and determination of
how the oxidation state of blood is altered.
Within conventional chemistry, there are two forms of bonding of
atoms that occur: ionic and covalent. Ionic bonding means that
electrons are transferred from one atom to another.
Covalent bonding means that the
electrons are shared between atoms. Bonding is important because the
physical properties of a substance are generally entirely different
depending upon the type of bonding that exists. Therefore, if you
know what type of bonding is occurring within a molecule or
substance, you can likely determine quite a bit about the physical
properties and behavior of the substance.
In our case, this is not an academic
exercise and we do not have a choice; we need to learn as much as we
can about the properties of the blood and how it interacts with the
rest of the body. Science is more meaningful is we can give value
and application to our studies and in our current situation, our
very lifeblood and welfare depends upon this pursuit.
Consider taking some time to learn about
the chemistry and biochemistry that is involved here and we will all
be the better for it.
The following are simple illustrations of both ionic and covalent
bonding:
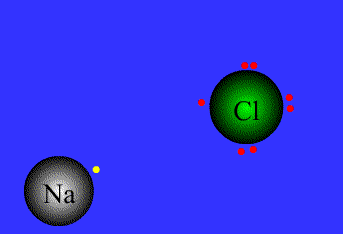
An example of ionic bonding. The transfer of electrons characterizes this bond form. Source:
Northeastern Oklahoma A&M College
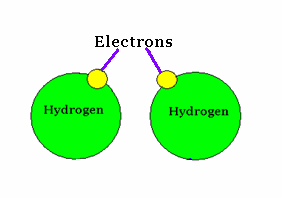
An example of covalent bonding. The sharing of electrons characterizes this bond form. Source :
Mr. Wolgemuth GHHS Science Web Site
Next, a brief word on polar covalent bonding: Polar covalent bonding
is a variation on the covalent bonding theme shown above.
In the example above on covalent
bonding, the forces on the electrons are symmetrical. When different
types of atoms join together (as shown below) vs. atoms of the same
type (as in the two hydrogen atoms shown above), the forces between
the electrons are not necessarily symmetrical. This asymmetry of
forces between shared electrons is referred to as a polar covalent
bond.
A simple example of polar covalent
bonding, i.e., the water molecule, is shown immediately below.
These three types of bonds: ionic,
covalent and polar covalent cover most of the ground of conventional
and introductory discussions of bonding of atoms within chemistry.
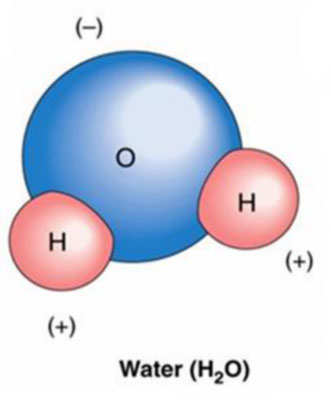
An example of polar covalent bonding. The asymmetric sharing of electrons and unequal distribution of
charge characterizes this bond form. Source : Zendarie : Biology One Step At a Time
We, however, in our journey of understanding the nature of iron
bonding within blood, are not allowed to stop here.
We will find that the three bond types
above do not tell us what we need to know about the way in which
iron is bonded, or "held" within the blood. There is indeed a fourth
type of bonding that we will introduce, and we will find that it is
different, unique, interesting and important to know about when it
comes to understanding what is happening within our blood.
The bond type that is pertinent to our
need to know is called a "coordinated covalent bond".
The coordinated covalent bond is an interesting animal, as it does
not fit in very well with any of the conventional explanations of
bonding listed above. What has caught my interest is that the
coordinate covalent bond is not introduced in the forefront of
chemistry education, but from my vantage point, it can easily end up
being a most important form of bonding to know about.
It seems to me that one of the easiest
ways to attempt to visualize a coordinated covalent bond is to
imagine at atom being "held" or "suspended" or surrounded by
electrons, the forces of those electrons keeping the bond in place.
Let us get the formal definitions, and then go to work with an image
that can help us to understand this unique form of bonding.
Here are three definitions to work with:
To start:
"A coordinate covalent bond is a covalent bond in which one of the
bonded atoms furnishes both of the shared electrons".13
Also:
"A particular type of covalent bond is one in which one of the atoms
supplies both of the electrons. These are known as dipolar (or
coordinate, semipolar, or dative) bonds."14
And:
"A covalent bond occurs when one atom contributes both of the shared
pair of electrons. Once formed, there is no difference between a
coordinate bond and any other covalent bond."15
And lastly, for the person in greater need, here is a more detailed
online definition16 and description of the coordinate covalent
bond.16
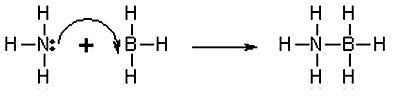
An example of coordinate covalent bonding. This is called a Lewis diagram and it shows the arrangements of the
electrons in the outer shell of the atom and how they are "shared"
or coordinated. Source: New World Encyclopedia: Covalent Bond
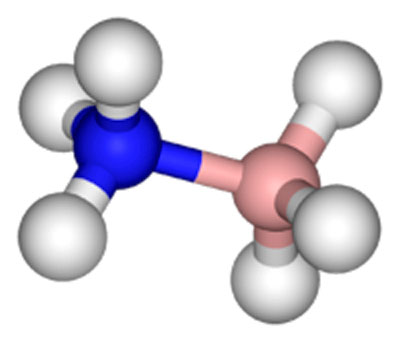
A three-dimensional model of the coordinate covalent bond shown to
the left.. Source: New World Encyclopedia: Covalent Bond
Now let us try to give more meaning to what the coordinate covalent
bond entails.
The images above depict one of the
simpler presentations of a coordinate covalent bond. Both images are
different views of the same bonding process. What the picture shows
on the left is that instead of one electron being shared by each
atom (in this case, Nitrogen and Boron) to form a shared pair, BOTH
electrons are donated by the Nitrogen atom and none by the Boron
atom to form the bond.
The end result is the same as in a
regular covalent bond, but the process by which the bond was
achieved differs from a normal covalent bond.
The reason that this type of bonding is
important is that many types of new and fundamentally important
"complexes" or chemical structures can be formed. Our blood
structure is one such example.
Many of the complexes that are formed in
this way involve the bonding of a metal atom (e.g., iron) with
surrounding molecules, and this leads us directly into our
discussion of the blood and the hemoglobin (or
heme) molecule.
The formation of what are called
coordination complexes or coordination compounds, very often with
metals at the center of the structure, is one of the most important
practical branches of chemistry. It is necessary for us to
understand coordination complexes in order to understand how the
iron in our blood bonds to oxygen.
And so now that we are in the thick of
it, on we go...
5. The
Structure of the Heme Molecule and the Role of Ligands
We are now in position to become more familiar with the detailed
structure of blood.
Our interest will be centered on
hemoglobin, and in even greater detail, upon what is known as the
heme molecule. Hemoglobin is an iron containing protein within red
blood cells. Hemoglobin is the molecule that transports oxygen.17
It is the iron of hemoglobin that binds
to oxygen.18
Heme is one of four subunits within hemoglobin. Each
heme group has an iron atom at its center, and therefore each
hemoglobin molecule can bind to four molecules of oxygen (O2).19 Our
primary interest will be in the heme group, as it is where the
oxygen carrying capacity exists. Here are a couple of images to
familiarize the reader with the overall structure of hemoglobin and
the heme group.
Subsequently, we will examine the heme
group in even greater detail along with the bonding process.
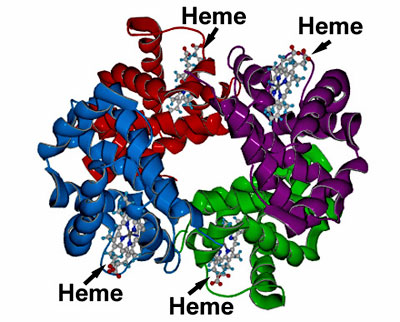
A generalized model of the hemoglobin molecule. Notice the four subunits of heme within the hemoglobin molecule;
this is where the iron atom exists that can bind to oxygen. Source: Washington University, Department of Chemistry
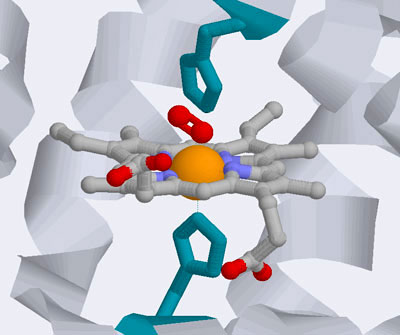
A closer view of the heme group. The iron atom (orange) resides in the center of the heme group. The
oxygen (O2) molecule is in red above the iron atom. We
will examine this structure and bonding process in greater detail
below Source : Wiley : Biochemistry
The type of bonding that allows the heme group to exist and to bind
iron to oxygen as shown above is the coordinated covalent bonding
that has been introduced previously.
This type of bonding allows the
formation of a multitude of metal complexes, and the heme group is
an example of one such structure that incorporates a coordinated
metal complex. These metal complexes and the unique type of bonding
they incorporate are have a special importance in biochemistry and
in blood.
Let us now look at the heme group in
even greater detail to understand the molecular structure further:
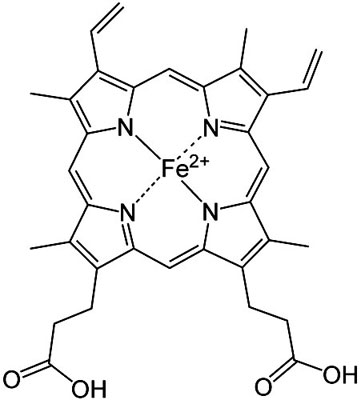
The heme group, consisting of an iron atom in the Fe2+ state,
surrounded by four nitrogen atoms bound with coordinated covalent
bonds. The iron must be in the +2 state to be able to bind to
oxygen.. Image source: Wikipedia
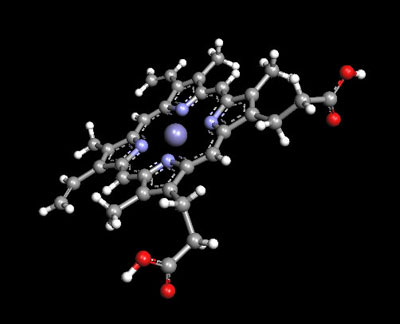
A three-dimensional model of the heme group, with the iron (II) atom
at the center surrounded by the four nitrogen atoms. This type of
structure is known as a porphyrin. One of the best known porphyrins
is heme, which is the pigment in red blood cells. Source: Argus Lab
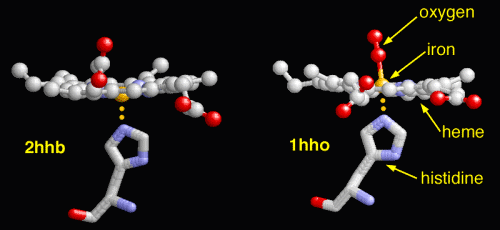 |
The dexoxygenated heme molecule (model) shown with oxygen atoms removed (red) |
The oxygenated heme molecule (model) shown with oxygen atoms attached. |
The heme group consists of an iron atom in the center of a ring
structure, termed a porphyrin.
The porphyrin includes the central iron
atom in the +2 oxidized state and is surrounded by four nitrogen
atoms with coordinate covalent bonds. The upper two photographs of
this sections show this structure in both a planar view and a
three-view.
The coordinate covalent bonds, as discussed earlier,
allow the transition metals such as iron to bind to a host of
varying molecules.
This type of structure is also that
known as a
chelate, where a central atom is bound to surrounding
molecules or structures (termed ligands). A great variety of
molecular structures with the transition metals can occur with this
unique and more complex bond type, i.e., the coordinated covalent
bond.
The lower photograph shows two additional aspects of the heme
molecule and the bonds that it makes within. These include the
histidine (an amino acid) structure and the oxygen molecule. The
oxygen molecule is at the heart of the discussion here.
The left photograph within the pair
shows the oxygen molecule removed from the heme group and the right
photograph within the pair shows the oxygen bound to the Fe2+ atom.
The iron must be in the Fe2+ state for the oxygen to bind; transport
of oxygen is a vital and crucial function of the blood within the
human body. If the iron in the blood is changed to the Fe3+ state,
the bonds to oxygen are broken and the blood is then known as
deoxyhemoglobin.
The primary cause of change in the
oxidation state of an atom is from an oxidizer; some of the best
known oxidizers include the hydroxyl radical, ozone, peroxides and
bleaches. 20
Oxidizers exist with the human body to
some level naturally. There is a body of evidence available in the
literature that will demonstrate that excessive exposure to
oxidizers within the body can be detrimental to human health.
Oxidizers produce free radicals, which are highly reactive molecules
that can "wreak havoc within the living system". 21
Some of the most important free radicals
in biology are the superoxide anion (O2-), peroxide (O2-2) and the
hydroxyl radical (OH).22
It will become apparent that the change in oxidation state of iron
from Fe2+ to Fe3+ in sufficient numbers within the blood is
generally detrimental to the blood and human health.
It will become
equally apparent that this change is especially beneficial to the
growth of the organism and filamentous biological growth structures
that are characteristic of
the Morgellons condition.
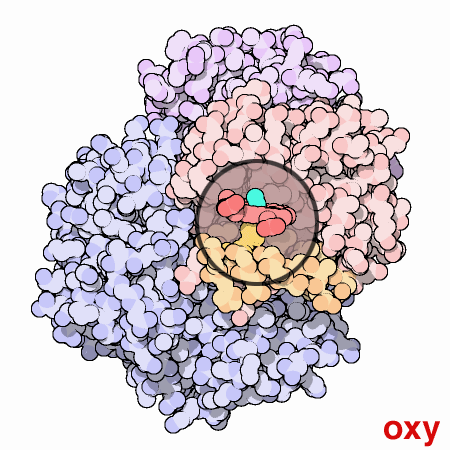
An animated view of the change between the oxygenated and
deoxygenated states of the blood. Correspondingly, this results is a
shift between the Fe2+ oxidation state of iron and the Fe3+
oxidation state of iron in the blood.
Source :
Protein Data Bank
6. Qualitative
Chemical Analysis of the Oral Samples
Two Methods to Verify the Existence of Ferric
Iron
We are now in a position to better understand and interpret the
results of more direct laboratory analysis.
It will be found that there is
essentially little difference between the direct human filament
samples that are under examination and those that result from the
culturing process demonstrated repeatedly on this site.
At this point we will deal directly with
human oral filament samples as the chemical reactions that are
common to both forms are now better understood.
It has long been observed that extended exposure (e.g., three
minutes +/-) of the oral gums to red wines produces in many, if not
most, individuals a purplish filamentous mass than can be expelled
and further analyzed.
This discovery is fully credited to Gwen
Scott, N.D. as originally reported several years ago. 23,24
It is claimed by some individuals that
this mass is of a precipitate form and that it is a natural reaction
between red wines and saliva. The reaction referred to is valid and
has been studied as well. However, the statement as it has been made
is entirely false as it refers to the samples under examination. The
sample under examination is of a filament form, and it is not a
precipitate.
The sheer volume of material that can be expelled, let
alone the examination of the material, is sufficient to dispel the
false and diversionary claims.25
The chemistry of this rather dramatic reaction of filament
production and coloration has, prior to this study of the last
several months, been unknown. This is no longer entirely the case,
and the subject will be introduced again later in this paper.
For now, suffice it to say that a most
significant chemical reaction and filament production does take
place, and the discovery can be regarded as serendipitous and
fortunate to the studies that have been made.
Given that such a reaction and production of mass does occur, this
study has now examined the material in greater depth from a
qualitative chemical perspective.
It has also been known for some time now
that the filaments do break down and undergo chemical transformation
when exposed to a solution of sodium hydroxide (lye) and heat.26
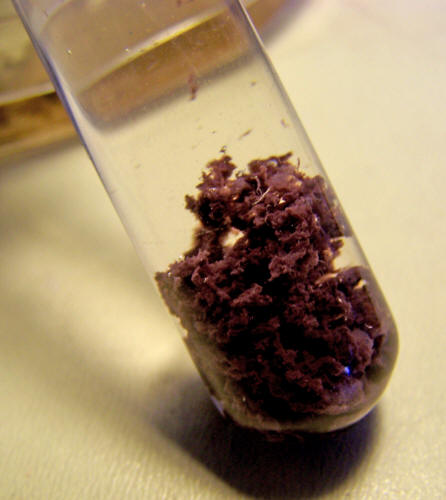
An oral sample filamentous mass produced from extended exposure of
the mouth gums to red wine. The sample has been repeatedly rinsed
and decanted in distilled water. The purplish color and microscopic
filaments are characteristic of the sample.
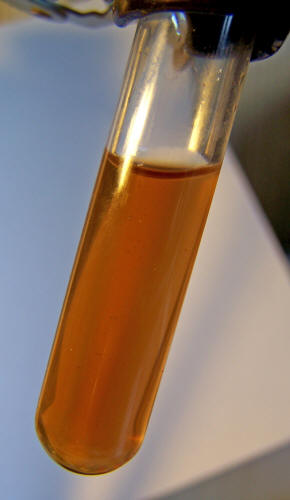
The oral sample after it has been subjected to a process of
alkalizing, heating and filtration. The sample is treated with
sodium hydroxide (lye) in solution and heated to the boiling point.
The solution is then filtered and produces the colored solution
above.
Please recall that the color of the ferric ion (3+) is
usually yellowish to brownish and that the color of the ferrous (2+)
ion is generally more greenish in color. This result of this process
indicates that the ferric (3+) iron form is a candidate for further
investigation in this qualitative analysis.
The photographs above show the original sample (to the left) and the
sample after processing with alkali, heat and filtration (right).
The solution on the right is also suitable for spectrophotometric
analysis, as shall be discussed later. At this point, we will be
concerned only with qualitative chemical reactions.
It is already known that the sample in the solution form prepared
immediately above fails a test for the existence of Fe2+ and Fe3+
ions.
This has been shown with similar results
for the culture form of this study earlier in this paper. This
result does not mean that iron does not exist in the solution, only
that it does not exist in disassociated ionic form.
The reason that the effort has been
expended to understand the various types of chemical bonding is that
because unless we know in what form a substance exists in solution
we may not be able to detect it with common testing methods.
This is the reason that an understanding
of coordination complexes and coordinate covalent bonding is so
essential; we must press the problem further and examine all options
with respect to the possible existence of iron forms within the
solution.
The following three factors are thought
to be relevant in the examination of the reaction of the oral sample
solution with a copper sulfate solution:
First One of the types of chemical reactions is called a single
displacement reaction. In a general way, this reaction has the
form:28
A + BC -> B + AC
or
A + BC -> C + BA
...and if A is a metal, A will replace B to form AC, provided A is a
more reactive metal than B.
Second Another relevant topic here is what is called the activity series of
metals. Some metals are more reactive than others, with water or
acids and the activity series of metals lists that reactivity in a
tabular form.
For example, potassium, calcium and sodium are highly
reactive metals with water, iron and nickel are moderately active,
copper and silver are of very low reactivity, and gold and platinum
are inactive.
Here is an example of an activity series table:27
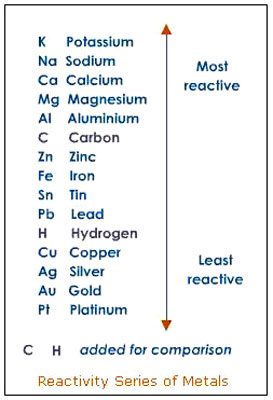
Source
It will be found that a metal higher on the list will replace a
metal that is in ionic form and is lower in the list.
Third Another helpful known reaction is that iron ions (+2 and +3 states,
respectively) in solution with sodium hydroxide will form ferrous
(+2) hydroxide, a green precipitate - Fe(OH)2 - and ferric hydroxide,
a brown precipitate - Fe(OH)3 - respectively.
The first chemical reaction that becomes of interest to study is the
oral sample solution above when mixed with copper sulfate. It will
be found that a reaction does occur, and the reaction is that a
brown precipitate forms. This indicates that we are likely to have
formed ferric hydroxide and this gives us another hint that we may
be encountering iron within a +3 oxidation state within the original
solution.
The issue is complicated, however, by
the fact that we know the iron is apparently not in ionic form.
This would suggest that we are dealing
with iron in a coordination complex of some type, where the iron is
bound to an unknown ligand. There are still uncertainties in this
problem, but it appears that the copper sulfate is somehow a factor
in releasing the iron from a complex form (presumably affected by
the activity series above) so that it can combine with the hydroxide
ion to form ferric hydroxide.
A proposed reaction is somewhat akin to
the form:
Fe3+X + Na+ + OH- + CuSO4 + H2O ->
Fe(OH)3 + Cu2+ SO42- + Na+ + H2O + X
...where X is an unknown ligand that is
attached to the iron ion.
The resulting reaction has been tested
further for copper and sulfate ions, respectively, and the results
are positive and are therefore consistent with the above reaction.
An alternative proposed reaction is of the form:
[Fe(H2O)6]3+
+ Na+ + OH- + CuSO4 -> Fe(OH)3 + Cu2+
SO42- + Na+ + 6H2O
...in which case the ligand is water and
involves coordination with the hydrated ferric ion.
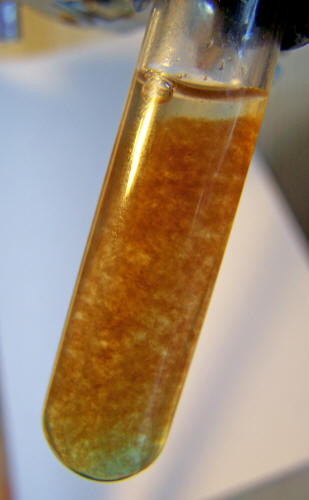
A reaction of the oral sample solution with copper sulfate. A brown
precipitate forms. A postulated identity of the precipitate is that
of ferric hydroxide which contains iron in the 3+ oxidized state.
The proposed ligand form is one question that will need to be
addressed further.
In the interim, the important question to pursue
is whether or not the precipitate is consistent with a ferric (vs.
ferrous) hydroxide identity. To further test the proposal of ferric
hydroxide as the precipitate, it will be found that ferric hydroxide
is soluble in citric acid. 29
It is also known that ferrous hydroxide,
when dissolved in citric acid, will turn the solution green
(characteristic of the ferrous ion). Ferric hydroxide, when
dissolved in ctiric acid will turn the solution to a brownish color
(characteristic of the ferric ion). This test has been conducted and
the result is positive, the precipitate is soluble in citric acid
and the resulting solution is brownish in color.
This further solidifies the proposed
identity of the precipitate as that of ferric hydroxide.
A second method of verifying the existence of the ferric form of
iron within the oral filament sample has been established.30 This
method involves the reduction of the Fe3+ iron state to the Fe2+
state using ascorbic acid, and then testing for the existence of the
iron in the Fe2+ state.
The steps of the process are:
-
The oral sample must be extracted
with the red wine and the test conducted promptly; this is a time
sensitive process that has been created.
-
The oral filament sample is rinsed repeatedly in clear water and
decanted until the final mass is in clear distilled water.
-
The sample is treated with sodium hydroxide and' heated to the
boiling point and then filtered. The solution will be brownish in
color as described earlier.
-
The solution is then treated with ascorbic acid. Ascorbic acid is
a strong reducer (anti-oxidant).
-
The solution is then centrifuged.
-
The clear solution that results from centrifuging is separated
and placed in a separate test tube.
-
A test for the Fe2+ ion is conducted using
(1,10) phenanthroline.
The test results are positive. This test demonstrates the reduction
of existing iron in the Fe3+ state to the Fe2+ state.
In the reference cited, it will be noted that potassium ferricyanide
is used in the reaction.
This experiment introduces the role of
another ligand that will be discussed in more detail later, and this
is the cyanide ion. It will be seen that varying ligands form
complexes with the transition metals.
This is one of the many
reasons we must familiarize ourselves with coordination chemistry
and coordinate covalent bonds to understand how this organism
interacts with the body.
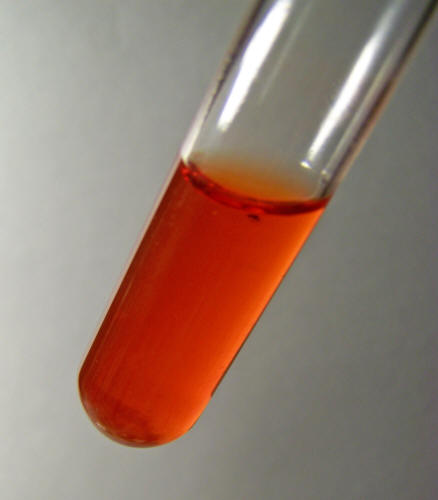
A positive test for the existence of the ferrous ion after reduction
by ascorbic acid using (1,10) phenanthroline.
7. A Method to
Extract the Oxidized Iron from within the Filament Growth Structure
A third and final method of verifying the existence of the ferric
form of iron within the oral filament sample has been established.
In this case, the iron itself in an
oxide form has been extracted directly from the oral filament sample
using electrolysis. The method is both simple and effective. Many
metallic salts, when subjected to electrolysis, liberate a gas at
the anode and deposit the metal in pure form at the cathode.
31,32,33,34
Presumably this can apply to certain
transition metal (e.g., iron) complexes as well and as evidenced by
the results obtained. The method used is to apply a current to the
oral sample solution directly.
Voltage is applied at 6 volts for
approximately 8 hours of time. The current in the solution has been
measured at 0.7 mA. The electrolyte is sufficiently decomposed at
the end of that period. The metallic compound is collected and
heated and dried at the end of that period. It appears as though the
bonds in the compound are quite strong as the compound is only
mildly soluble in strong acids such as hydrochloric and sulfuric
acids.
The compound reacts vigorously to
hydrogen peroxide as shown below in the video segment. The reaction
shown involving the decomposition hydrogen peroxide to oxygen and
water is an established and known catalytic reaction (in the same
genre as Fenton's reaction).35,36
The results of all qualitative tests indicate that a ferric (3+)
iron is a highly significant component of the growth structure and
organism development. It is also presumed at this stage of the
analysis that the iron exists primarily within a transition metal
coordination complex with ligand structures that require further
analysis and identification.
An additional discussion on the ligand
aspect of this study will follow.
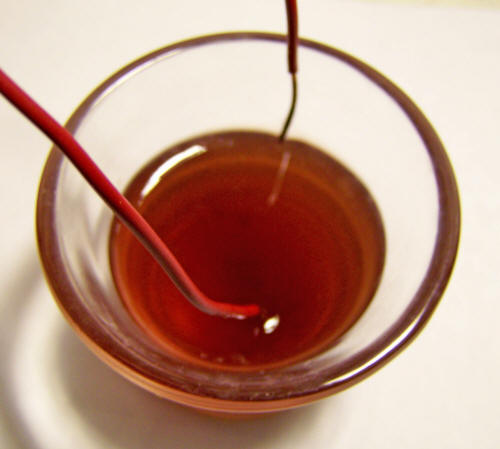
Pre-electrolysis of the oral sample solution.
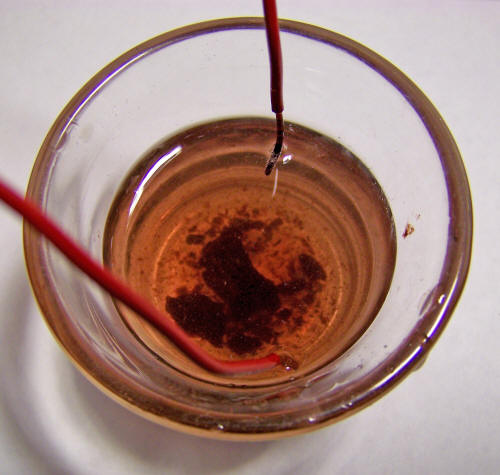
Post-electrolysis of the oral sample solution.
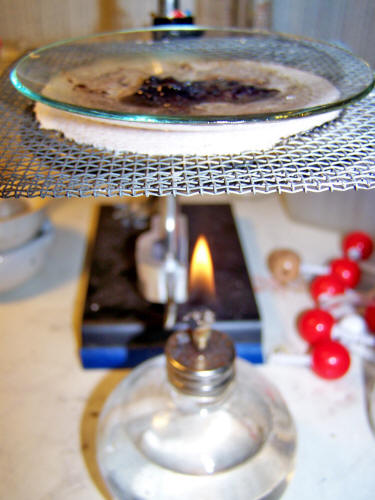
Drying the metallic residue from the electrolytic processing of the
oral sample.
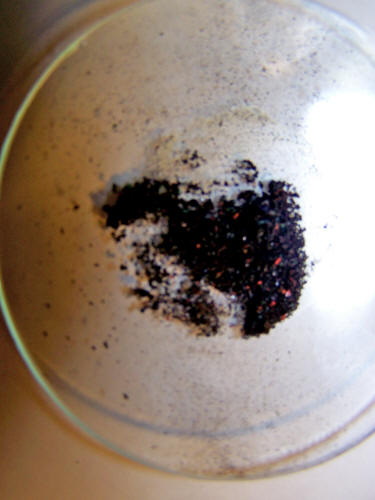
The final iron oxide (ferric oxide) compound result obtained
directly from the oral sample through electrolysis.
Ferric Oxide Compound and Hydrogen Peroxide Chemical Reaction: This is a catalytic reaction that does not result in a change in the
iron oxide form or mass. Magnification approx 75x.
8. A
Discussion of Ligands
Let us talk about ligands for a moment.
Remember that a coordination complex is
formed with a metal atom at the center of the complex surrounded by
atoms that donate electrons to form the coordinate covalent bonds.
These donor structures are called ligands. The heme group that we
discussed was a representative example of such a coordination
complex, with the iron atom in the center of the ring with nitrogen
atoms surrounding the iron.
We also have a histidine (amino acid)
group attached to the heme and then the oxygen molecules at a sixth
position in the complex. We have also seen that the oxygen molecules
can come and go within the complex depending upon the state of the
iron in the center of the complex.
Please review some of the images and
discussion above if you would like to recall this discussion.
It now is becoming more apparent to us why we must understand the
specific molecular structure of the hemoglobin molecules (especially
the heme group within) and' of the transition metal (notably iron)
coordination complexes within the heme group.
It is also equally important that we
must learn more about the impact of "ligands", as ligands are the
atoms or structures that bind to the metal.
Coordination chemistry
seems to be a bit more involved than conventional chemical study as
the bonding structures are highly varied and more difficult to
predict. But the necessity exists here, for what binds to the iron
(i.e., ligand) that has been altered (i.e., oxidized) is going to be
extremely important in understanding the impact or predicted impact
upon the body.
For instance, the importance of this
topic can be stressed with the following:
"Metal and metalloids are bound to
ligands in virtually all circumstances... Ligand selection is a
critical consideration in many practical areas, including
bioinorganic and medicinal chemistry, homogeneous catalysis, and
environmental chemistry." 37
Therefore, we will need to understand
ligands and coordination complexes in more detail to help us get out
of the mess that we are in. Please engage yourself in that process
as it appears that it will become very important in understanding
the human health effects that are in place as we speak.
An introduction to this topic involves what is called the "spectrochemical
series". Fortunately there is a knowledge base available to help us
understand what ligands (or chemical structures) are more likely to
attach to metal ions, such as iron, than others are.
Three fields of study that are helpful
in this regard are:38
-
The Spectrochemical Series
-
Ligand Field Theory
-
Crystal Field Theory.
The latter two topics are more advanced fields of chemistry study
and can only be briefly mentioned in this report.
The latter two subjects, Ligand Field
Theory and Crystal Field Theory, help us to understand how the
spectrochemical series has developed. In this paper, we need to
focus on this end result to start with and to at least become
familiar with the spectrochemical series.
The spectrochemical series is a ranking of ligands, according to
what are called weak field ligands and strong field ligands. Both
abbreviated and longer form tabulations of the spectrochemical
series exist depending upon the level of investigation.
An example of an abbreviated
spectrochemical series is as follows:39
I- < Br- < SCN- < Cl- < F- ≤ OH- ,
ONO- < OH2 < NCS- < NCCH3 < NH3 , py < NO2- < CN- , NO , CO
weak-field ligands
strong-field
ligands
Recall that the most important feature of a coordination compound is
the donation of a pair of electrons by the ligand (i.e., donor) to
form a coordinate covalent bond with the metal.
As a first generalization, softer metals
generally prefer bonds to weak-field ligands and harder metals (e.g.,
iron) are more likely to bond with strong field ligands. 40
It can also be cited that the cyanide
ion and carbon monoxide would be expected to have a rather strong
affinity for the ferric (3+) ion.41 This type of
relationship will be critical in our understanding and future
direction of research in relation to the altered blood that has been
identified in this report.
Separate research from a variety of
sources 42,43 has also disclosed the following list of
candidates ions or molecules to consider as potential ligands to the
oxidized iron (+3) atom (this list will overlap with the
spectrochemical series):
CO, CN-, NH3, H2O,
OH-, SO, NO2 S2- N3- NO2-,
Cl-, CH3COO
Please be aware that many of the ligands
under review above are toxic or interfere with biological processes.
As examples, the cyanide ion, azide ion
and carbon monoxide are each respiratory inhibitors to some degree.
Although an introduction to a significant problem related to oxygen
deficiency (methemoglobinemia) will be discussed later in this
report, much research remains to be tackled on the subject of
ligands and oxidized iron.
Please consider the support of this
research if you are so inclined.
9. Spectral
Analysis of the Blood and a Comparison to the Growth Spectrum
Extensive spectrometric analysis human blood is the original basis
for this report.
It was observed early on in the process
that the expected spectrum of normal hemoglobin was not being
observed using blood samples from a variety of individuals.
This
required establishing a "reference spectrum" for hemoglobin based
upon that of record and upon historical public data. Please review
the previous paper entitled Altered Blood 44 for an
introduction to the situation at hand.
This paper remains current and accurate
with the information acquired and analysis completed thus far.
The graphs below show the general nature of the predicament.
The
purpose of this section will be to summarize only briefly the work
of several weeks of observation and investigation of sample
hemoglobin vs. the reference spectrum that has been established.
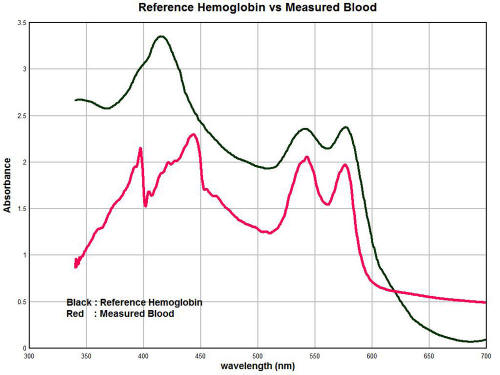
The black line is the reference spectrum for hemoglobin that has
been established through examination of the literature and available
tabulated data. The red line is the average spectrum of
approximately ten individuals over the same visible light wavelength
range.
Clearly there is a significant
difference. A salient change that can be identified is the
appearance of two strong peaks in the vicinity at approximately 397
nanometers (nm) and 448 nm.
These strong peaks substitute themselves
for the prominent expected peak at approximately 414 nm. The
magnitude of absorbance can vary strongly according to concentration
levels so the magnitude of the peaks so there must be some latitude
given to the conclusions related to that aspect. Nevertheless, in
general we see that the magnitude of absorbance is strongly reduced
in the measured spectrum vs. the reference spectrum, especially in
the range of 300-350nm.
The difficulty then becomes to explain these sharp differences
between the spectrums.
We can begin this analysis by examining
the spectrum of the cultures as they have been developed from oral
samples and examples of this work are shown below.
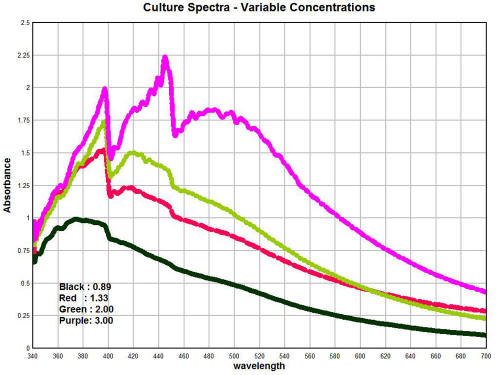
The graph above shows the spectrum of the culture as developed from
oral samples.
The primary variable within the graph is that of
concentration. These graphs show the importance of concentration and
how it can affect the geometry of the spectrum. It can be seen in
general that an increase in concentration causes a corresponding
increase in the absorbance; this is an expected consequence of
Beer's Law is it relates to spectroscopy.
It is also of special interest to note
that with sufficient concentrations that a second peak appears at
approximately 448nm; this peak was simply not observable at low
concentration levels.
A calibration curve for the
concentration of the culture mass has been developed from this work.
A fair amount of culture mass is required to produce the highest
concentration levels shown; these details of solution preparation
can be described further as time progresses. It has already been
reported that the solutions are produced primarily with the use of a
strong alkalizer (sodium hydroxide) and heat; this method is
successful in breaking down the filament nature of the culture to a
sufficient degree.
There is an extremely important observation that is to be made from
these graphs shown here. It is that the geometry of the peaks of the
culture, as it has been developed from oral filament samples, is
essentially identical to those deviations that are reported in the
measured hemoglobin spectrum shown immediately prior.
Within the culture spectrum, we see
corresponding strong peaks at approximately 397nm and 448nm, exactly
the same peak structure that is apparent in the hemoglobin spectrum
under measurement in a sample of individuals.
This suggests, in a highly logical and
sensible fashion, that we should consider looking at the growth of
the organism as a significant factor on the alteration of the
hemoglobin spectrum as it is being directly measured.
The next issue of importance is to identify what is the underlying
nature of the culture, or organism, spectrum. A spectrum in itself
is valuable for its uniqueness, but the interpretation of the
underlying spectrum is a much more involved affair. It involves a
body of knowledge than can represent a profession it is own right.
Some of the factors that affect the
manifestation of the spectrum include the elements involved, the
types of molecular bonds involved and the energy states of those
atoms or molecules.
I do not profess to know that science to
that level of detail to immediately be able to interpret a visual
light spectrum at the elemental and atomic bond level; by the same
token the subject matter is not entirely foreign to me at this stage
of study.
The process of investigation on my end is too laborious and time
consuming to describe here, and the extensive time and effort
extended is to be summarized in a succinct manner for your benefit
In that protracted process, the spectrum of iron salts has also been
examined in some detail.
Suffice it to say that the spectrum of
the ferric ion (3+) in solution matches remarkably well with the
spectra culture and oral sample spectrums, especially in the range
of 300 - 475nm where the deviations reported above most strongly
occur. This was indeed the discovery that has motivated the
intensive focus on iron with respect to this particular growth form,
or "organism", as it were.
It is also the very reason why the
qualitative chemical studies described above were developed.
I have attempted to approach the problem
from numerous angles to seek a consistent resolution to the problem.
At this point, it seems fair to claim that such a consistent
resolution has been reached.
The role of iron in the oxidized state
(3+) and its importance in the growth of the organism, from this
researcher's perspective, appears to be positively established.
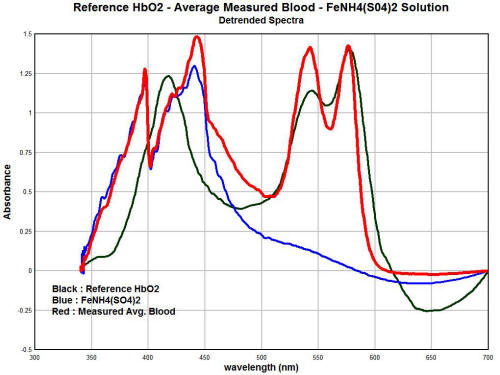
The final graph in this section shows the degree of overlap that is
occurring between the hemoglobin spectrum as it is being measured,
the spectrum of the oral and culture samples, and the spectrum of
the ferric ion (3+) in solution.
The degree of similarity and overlap is
actually quite remarkable and further solidifies the arguments that
are presented within this paper.
In these graphs, the trends of each individual spectrum has been
removed. This has the advantage of essentially normalizing the
magnitudes of the graph so that we can focus on the degree of
similarity of the absorption peaks. We have three different spectra
shown here. The red line is the average measured spectrum of
hemoglobin from a sample of approximately ten individuals.
The black line is the spectrum of the
"reference hemoglobin" as it has been obtained from the available
public sources. The blue line is the spectrum of a dissolved ferric
(3+) salt, specifically iron ammonium sulfate.
There are some important observations to
me made here that reiterate the degree of similarity that has been
established prior. We see a very close match between the spectrums
of the measured hemoglobin spectrum and the ferric ion (3+) in the
lower half of the visible spectrum (350 - 475nm).
This strongly suggests that the ferric
(3+) form of' iron is intimately involved in the deviation of the
measured hemoglobin spectrum from the reference hemoglobin spectrum
It is indeed the basis of this thesis, as the body of evidence
established now demonstrates that this is exactly the case.
Secondly, we see that the magnitude of the spectrum of the ferric
ion drops off radically in the upper half of the spectrum, i.e., 475
-700nm. This means that we would expect the ferric ion to have much
less influence upon the spectrum of hemoglobin within that range.
This is also exactly what we find. We
notice that the reference hemoglobin spectrum and the measured
hemoglobin spectrum actually compare reasonably well in the upper
half of the visible light spectrum. This spectral analysis
establishes the case quite strongly, therefore, that the ferric (3+)
ion form plays a prominent role in the alteration of blood as it has
been measured from several individuals.
It is at this point that we must recall
that deviation of the iron in the blood from the normal state of
Fe(2+) to that of Fe(3+) presents serious health consequences.
The
most important of these is the inability of iron in the ferric state
within blood to bind to oxygen.
This leads us to the next topic below.
10.
Methemoglobinemia and Hypoxia
Now that certain results have been established, we must anticipate
and begin to deal with the consequences of those results, should
they be proven to be true.
To reiterate, these results present
themselves in two primary forms:
-
The evidence indicates that the growth form central to the Morgellons condition utilizes iron in a ferric (3+) state for its
own growth, development and sustenance.
-
The evidence indicates that human blood is altered significantly
as a result of the presence of the organism within the blood. This
alteration encompasses a partial change of the oxidation state of
the iron within the hemoglobin from a ferrous (2+) to a ferric (3+)
state. Iron in the ferric state (3+) within hemoglobin is unable to
bind to oxygen.
If these findings are true, we are required to pursue the next
logical line of investigation, i.e., diminished oxygen carrying
capacity of the blood.
There is a known medical condition for this
change within the blood, and it is called methemoglobinemia.
Methemoglobinemia is the transformation of normal hemoglobin (oxyhemoglobin)
to a deoxygentated state. Methoglobinemia is caused by the oxidation
of the ferrous ion (2+) to the ferric state (3+). Ferric iron is
chemically useless for respiration. 45
Methemoglobinema can exist at
varying levels, and is usually expressed as a percentage of the
total hemoglobin of the blood. It is a normal state to have
approximately one to two percent of methemoglobinemia (ferric ion)
in the blood.46
Mild methemoglobinemia, on the order of 2-10%, is generally well
tolerated by individuals and usual presents no obvious or apparent
symptoms.47
There is, nevertheless, a diminished capacity of the
blood to carry oxygen at this stage and the effects are not to be
dismissed as we shall discuss further. At levels from 10 -15%,
cyanosis will occur with the skin taking on a blue/gray cast or
appearance. Higher levels still, e.g., above 20% can cause dizziness,
increased heart rate and anxiety.
Levels greater that 50% are
associated with breathlessness, fatigue, confusion, drowsiness.
Comas, seizures may also occur at this level. Methemoglobinemia at
70% or greater is usually fatal. 48
From the results of this paper, it the following hypothesis can be
presented. It it is accepted that the Morgellons growth form is
responsible for a partial alteration of the blood from a ferrous to
a ferric state, it follows that those with a more serious
manifestation of the condition may demonstrate a tendency toward
increased levels of methemoglobinemia.
Whether or not this is the
case is to be determined by the medical profession at some time and
place, however, initial investigative work on this proposal will be
presented within this report.
Although only a preliminary and
tentative analysis, one spectrometric/chemical analysis made has
indicated a potential level of an approximate 7% oxidation state
(3+) in the average hemoglobin measurement of this report. This
level would be without obvious visible symptoms as described
earlier.
This analysis requires further examination to substantiate
that finding.
Obviously there are many purported and claimed manifestations and
variations of the so-called "Morgellons" condition, and this paper
is not able to encompass that scope or debate. The work of this
researcher places a focus on what is perceived to be an originating
growth form as identified through several years of observation and
analysis of various sample types (primarily filamentous in nature.)
This paper will simply not have the capacity to discuss all of the
ramifications of diminished oxygen capacity of the blood; it will
have to suffice at this point to state that this process of
discovery must now begin.
Some occasional comments on the subject
will be presented as time and circumstance allow me. Degrees of
hypoxia and its effect upon cellular metabolism will also become a
point of investigation in our future. As a starter, please recall an
opening statement that all energy to the body is dependent upon
respiration.
Finally, to end this section for the time being, a visual
representation of the nature of methemoglobinemia (deoxyhemoglobin)
is repeated below for the reader's reference.
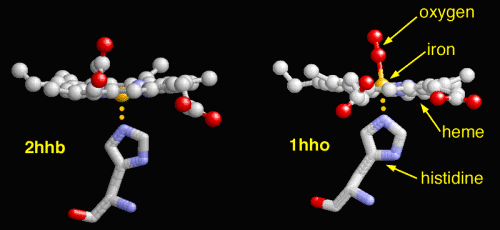 |
The dexoxygenated heme molecule (model) shown with oxygen atoms
removed (red) |
The oxygenated heme molecule(model) shown with oxygen atoms
attached. |
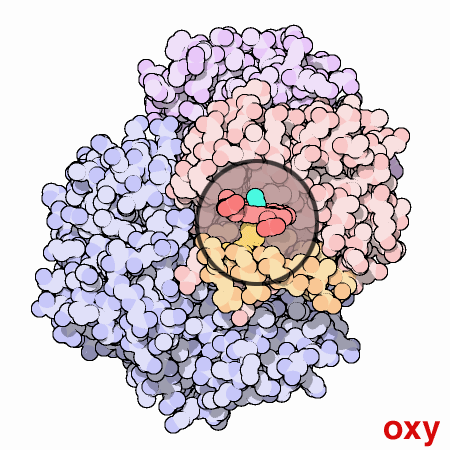
Source :
Protein Data Bank
11. Ionization
and Bond Disassociation Energy
The Cost of Oxidation
It requires energy to form molecules. 49
It requires energy to remove
an electron, i.e., oxidize an element or molecule.49 And it takes
energy to break bonds.50 What this means, in simple terms, is that
the theft of energy from our cells to serve the metabolic
requirements of a pathological organism comes at a price to our body
and our health. The removal of an electron is called the ionization
energy.
These are referred to as the first ionization energy, second
ionization energy, third ionization energy, etc. corresponding to
the removal of one, two and three electrons respectively.. There is
energy required to remove two electrons from iron in the elemental
state to the oxidation state of iron (Fe2+). This oxidation state is
the one that is most commonly found in nature.
To remove an
additional electron, and bring iron to the Fe(3+) state requires
even more energy. Oxidation essentially represents the stealing of
electrons from one element or molecule by another.
The first ionization energy for iron is 7.9 electron volts (eV)
(~760 kilojoules (kJ) per mole), the second ionization energy is
16.2 eV (1560 kJ per mole) and the third ionization energy is 30.6
ev (2960 kJ per mole). 51
What this shows us is that it takes almost
twice as much energy to remove the electron to change the iron from
the ferrous (Fe2+) state to the ferric (Fe3+) state as it did to
remove two electrons to change it from the elemental form to the
Fe(2+) state. From an energy standpoint, therefore, the oxidation of
iron referred to in this paper requires a relatively strong energy
investment.
To get some sense of what this energy level actually means, let us
translate what is happening in the blood to something more tangible
for us to visualize.
If we assume a 5% reduction in oxygenated
hemoglobin over a three month period (the approximate life cycle of
red blood cells), this will translate to an energy requirement of
approximately 3240 joules over this three month period.
(Humans have roughly 2.5E13 red blood cells; 280E6 molecules of
hemoglobin in each red blood cell; 7E21 molecules of hemoglobin in
each red blood cell; four heme molecules per red blood cell; approx.
2.8E22 Fe2+ iron atoms in the human body; at 5% oxidation 1.4E21
atoms in the Fe(3+) state ; .0023 moles of iron in the Fe(3+) state,
.0023(2960kJ/M - 1560kJ/M) = approx. 3260 joules over a three month
period.)
It takes approximately one joule of energy to raise an apple over
your head.
If these approximate calculations are correct, this would
be equal to raising roughly 3000+ apples over your head in a three
month period. This equates to roughly three dozen presses per day;
this is not exactly trivial since this energy expended should be
serving your own interests vs. the metabolism of a detrimental
pathogen. Regardless of the computations, the energy is stolen
energy.
It also takes energy to break chemical bonds. In this case, we can
at least look at the separation between the iron and oxygen atoms.
The bond dissociation energy for the iron-oxygen bond is 409 kJ per
mole.52
Again, even though we are making some approximations, this
leads to roughly another 940 joules of energy released in a damaging
manner if we assume the same three month period. Add lifting another
1000 apples to your detriment.
And lastly, it takes energy to form molecules. This brings up the
entire discussion of ligands again, as new molecules will form with
the oxidized iron, many of them harmful to the human body.
For
example, the ferricyanide complexes is one of the most likely
complexes to form from the altered iron, and it is toxic as well.
To
form that complex, or other complexes that result from the spectrochemical series, will require additional energy. From an
energy standpoint alone, you are doing bench presses on a regular
basis and your health is suffering in the process.
There is a cost for the oxidation of the iron in our bodies, and
that cost is to one's health.
12. Bacterial
Requirements for Iron in the Blood
For those patient enough to follow the course of this paper, it is
fair to state that significant efforts have been expended, from both
a laboratory and a research point of view, to demonstrate that
changes in iron and the utilization of iron in a pathogenic sense
are at the heart of the Morgellons issue, at least from the
perspective of this researcher.
The changes and impact upon the body
have been demonstrated and they will continue to be so.
For those
that are inclined to accept conclusions more readily from the
conventional literature, the following is provided from the section
entitled, Chemistry and Life, The Battle for Iron in Living
Systems: 53
"A bacterium that infects the blood requires a source of iron if it
is to grow and reproduce."
Recognition of the truth and simplicity of this statement may have
saved a great deal of time and effort, but this particular reference
was not found until the same conclusion was reached from direct
experience.
The time and effort has not been lost by any means, as
there is now a deeper understanding from whence this statement
comes. Let us now add some complimentary information to the direct
knowledge given to us from the statement above. First of all, it is
true that the work does not positively identify the sub-micron
spherical originating organism as a known or specific bacterium.
It
does, however, seem to be a most relevant consideration. At this
point, it is best to refer the reader to a prior paper that
expresses the proposition of essentially an "engineered" organism
54
that combines the prokaryote, eukaryote and archaea life forms. The
bacterial form is a subset of this larger life classification system
and the above statement holds as true and relevant to the work.
On a
more general level, we can delve into the question further and ask
whether bacterial forms are commonly involved in the consumption of
iron. The answer is yes.
From a variety of sources, we can only
confirm further the findings of the current research; the fact that
bacterial forms require iron for their survival is readily
verifiable:
"Like their human hosts, bacteria need iron to survive and they must
obtain that iron from the environment. While humans obtain iron
primarily through the food they eat, bacteria have evolved complex
and diverse mechanisms to allow them access to iron... Iron is the
single most important micronutrient bacteria need to survive...
understanding how these bacteria survived within us is a critical
element of learning how to defeat them."55
"Bacteria metabolize iron as a food source and release iron oxide as
a waste product...bacterial waste lowers pH."56
"The term iron bacteria does not refer to a specific genus or
species but rather to those bacteria in which reduced iron plays an
important role in their metabolism... A great variety of bacteria
can be involved in this process. The "true" iron bacteria are those
in which the oxidation of iron is an important source for their
metabolic energy. This group is most often associated with
filamentous or stalked forms..."57
"Bacterial requirements for growth include sources of energy,
"organic" carbon (e.g., sugars and fatty acids) and metal ions
(e.g., iron).....Nutrient Requirements: These include sources of
organic carbon, nitrogen, phosphorus, sulfur and metal ions
including iron. Bacteria secrete small molecules that bind iron (siderophores).
Siderophores (with bound iron) are then internalized via receptors
by the bacterial cell."58
"Siderophores are biosynthetically produced and secreted by many
bacteria, yeasts, fungi and plants, to scavenge for ferric ion
(Fe3+). They are selective iron-chelators that have an extremely
high affinity for binding this trivalent metal ion... The emerging
overall picture is that ion metabolism plays an extremely important
role during bacterial infections."59
"The ability of pathogens to obtain iron from transferrins, ferritin,
hemoglobin, and other iron-containing proteins of their host is
central to whether they live or die... Some invading bacteria respond
by producing specific iron chelators - siderophores - that remove
the iron from the host sources."60
"Iron is one of the most common elements in the Earth's crust and
forms a ready oxidation state. Bacteria use this as a source of
energy and as a means of waste disposal.. Iron metabolism is also a
significant part of bacterial virulence...It has been established
experimentally by injecting iron soluble compounds into test animals
with infections that adding more iron causes the bacteria to
thrive... Bacteria put out compounds, called siderophores, which
attract and bond free iron compounds by chemical processes; these
are then oxidized and excreted as a byproduct."61
"Iron (Fe) has long been a recognized physiological requirement for
life, yet for many organisms... its role extends well beyond that of
a nutritional necessity. Fe(II) can function as an electron source
for iron-oxidizing microorganisms under both oxic and anoxic
conditions and Fe(III) can function as a terminal acceptor under
anoxic conditions for iron-reducing organisms."62
"Given the role of free iron in creating DNA damage, it is
unsurprising that bacteria have evolved methods to scavenge
it... Despite the sophisticated biochemical and genetic strategies
that can be brought to bear upon bacteria, we still know remarkably
little about the physical mechanisms of iron transport, storage, and
regulation, and virtually nothing about iron trafficking and its
insertion into metalloproteins. These areas are ripe for future
work."63
As a parting comment within this section, there is a class of
siderophores produced by certain bacteria that bind in particular to
iron in the Fe(3+) state.64,65,66
These siderophores are called
enterbactin.
What distinguishes this class is an incredibly strong
bond to the iron (i.e., chelation) in the 3+ state, and it can not
be broken through normal physiological processes or with such
proteins as transferrin. This type of siderophore is usually found
in Gram-negative forms of bacteria.
Readers may recall that several
years ago gram stain tests were repeatedly performed on the
bacterial-like organism under study and discussion here. The results
of those tests were Gram-negative. Enterobactin and ferrichrome
therefore emerge as important targets of further research within the
iron dilemma.
The journey to the current state of knowledge has been a long one,
and for that matter, it has been unnecessarily long.
We can,
nevertheless, take some solace in knowing that some findings of
importance are before us. There is also now a stronger sense of
direction of what is required and what is to be done.
If you would
like to hasten this process, you have the opportunity to do so.67
13. The Oral
Filament and Red Wine Reaction Resolved
It has long been a mystery as to why there is such a definite and
visible reaction, especially of color, between the oral filament
samples and red wine or related solutions.
This mystery has now been
resolved with a combination of investigative chemical research and
the knowledge of iron changes in the body. The reason for the strong
reaction is the formation of a metal complex of Fe(3+) in
combination with the pigments found in red wine.
Once again, at
least some knowledge of coordination chemistry in combination with
transition metal characteristics proves fruitful. Grapes, red wine
and many related fruits or vegetables contain a group of pigments
called anthocyanins. A search of the literature will reveal that
iron, especially in the ferric state (Fe3+), will form metal
complexes with these pigments. 68,69,70,71,72
The color of many of
these metal complexes is often a deep purple, exactly that which is
known to occur in the combination of the oral filaments with the red
wine.
It is also of interest to learn that the molecular structure of the
complex, i.e, the combination of Fe(3+) with anthocyanins, has a
chemical structure with some similarity to that of ferrichromes.
Ferrichromes are a product of bacterial consumption of iron, and
they involve the formation of strong chemical bonds that tie up the
iron within a ferric metal complex.
It is the understanding of the chemistry of iron in its various
states along with the important but more complex branch of
coordination chemistry that has allowed us to understand the nature
of the ferric iron - red wine reaction.
This understanding provides
one further level of verification and confirmation of the change of
iron that occurs within the body as a direct result of the
pathogenic metabolism.
14. Some
Health Implications
The Value of the Holistic Approach to Medicine
For those that seek a pill to remedy the dilemmas of the Morgellons
"situation", you must seek elsewhere.
My work will not offer such a
simple path for you. The research of the past several years on the
bio-engineering issues has been a journey of education in health,
myself included. Out of this research I have developed a level of
respect for the holistic approach to medicine and for those who
practice it well.
Those who have this knowledge coupled with strong
foundations of chemistry, biology and physics will earn even greater
respect as they are likely to be our better sources for counsel.
Let us start with some of the controversy in language regarding the
issue of a "condition" vs. a "disease".
As the work indicates that
the general population is subject to the pathogenic forms under
study, it becomes even a more sensitive issue as we confront our own
involvement irrespective of our wishes or personal belief systems. I
will start this discussion with reference to a rather hefty tome,
Robbins Pathologic Basis of Disease.73
This book may not be bedside
reading for most of us, but in many ways it should be. It is a real
eye opener for the uninitiated. For now, let us introduce just a few
insights that this reference will provide to us.
First, what
pathology actually refers to, in the origin of the word, is
suffering. We can play with semantics all that we wish, but those
suffer in a biological sense will need to deal with the reality of
the terms pathogen and disease.
Cells, tissues and organs that
sustain injury are at the root of the study of pathology. Study the
textbook and reach your own conclusions as to the severity of
affliction. It is a diminishment to the reality and seriousness of
the issue if we classify the current situation as a "condition" for
our own personal palatabilities and psychological comfort.
It is
difficult to deny the classification of "Morgellons" as a disease or
as of pathogenic form if you look at the underlying mechanisms of
damage that have taken and are taking place. I may not please the
reader but that is not my purpose here; it is to confront and
comprehend the reality of our existence be it kind or brutal.
The next topic concerns what we must read to get started with our
education on pathology. Robbins' book is roughly 1500 pages long. If
we can digest even a portion of the first 40 pages, we have done
ourselves a great service.
It will be found that this introductory
section alone will spell out the majority of the specific mechanisms
and actions of injury to our health at the cellular level; this
foundation will underlie the remainder of the book which will go on
to address injury to further organs.
A knowledge of cellular injury
is crucial to our understanding of any disease and how it works its
damage upon the body. It is not especially relevant at this stage of
our discussion to single out the particular malady at hand;
understand the mechanisms of cellular damage in general and
tremendous progress can be made in the path to better health and
health understanding.
This particular book is more than 20 years old
and yet the level of knowledge on how disease damages the body is
evident, open and obvious to those that are willing to take a look
at it. This knowledge can be applied to any circumstance of illness
that I can foresee, past or present, including our current problems.
It will be to our benefit to invest this effort for what awaits us
as we learn to apply that knowledge.
A standard and comprehensive
book on pathology is at the very heart of medical knowledge and
application; those with a wholistic approach to medicine that seek
the source of a problem versus a prescribed band-aid deserve our
greatest respect and honor. This particular chapter of this paper
will never be completed as the pathways and connections within the
body never cease to amaze me.
I am an infant in these wonders myself
and must admit my own negligence with respect to the understandings
of physiology, disease and health.
In many ways, the course to
better health has been spelled out for us many years, even decades,
ago and it is our job to at least acquaint ourselves with the work
that has already been done for us.
This preparation established, let us at least briefly mention what
the four systems of damage (i.e., vulnerabilities) are to the cells
in our bodies through disease.74
These criteria form the very basis
of pathology:
-
Damage to the cell wall or membrane.
-
Aerobic respiration (i.e., oxygen based respiration) and the
production of energy within the cells.
-
The creation of enzymes and proteins within the cells.
-
Preservation of the genetic integrity of the cells.
My work indicates, at this point, that every one of these critical
factors underlying damage to our bodies is underway or is likely to
be underway within the mechanisms of the Morgellons pathogenic
forms.
It is much harder to prove that any one of them is not
involved than it is to make the case that they are in effect. If
this is to be accepted, the very core, foundation and definition of
"disease" is in full bloom here and it is only a diversion to avoid
that unpleasant reality.
The necessary job is to understand the
forces at work in great detail from a biochemical perspective and
then get to work on the solutions to the problems that they pose for
us a species and as a whole. The stakes are serious enough; make
your decision as to when and how your are going to become involved
in your own survival and those that follow.
Let us give some introductory examples or thoughts as to how and why
these factors are likely to be involved.
In terms of damage to the cell wall or membrane, the damage to the
red blood cell walls has been aptly documented. Please see the
previous paper entitled "A Mechanism of Blood Damage".75
In terms of aerobic respiration, it is also now clear that the
oxygen carrying capacity of the blood is expected to occur as a
result of the increased oxidation level (Fe3+) of iron with the
blood. Recall that oxygen does not bind to hemoglobin when the iron
is in the Fe (3+) state. If the oxygen carrying capacity of the
blood is diminished, the production of energy (ATP) is also expected
to be diminished.
With regard to enzyme (most enzymes are proteins) and protein
production within the cells, it is a fact that essentially all
cellular reactions that take place within the body require enzymes
for those reactions to occur.76
And, as an example of the tie
between iron and enzymes, approximately one-third of all enzymes
require metal ions 77 and iron is also an essential component of many
proteins and enzymes.78 If cellular metabolism is interfered with (i.e.,
the production of energy by the mitochondria) then the catalytic
reactions involving enzymes within the cells are disrupted.
Lastly, oxidation in the body produces free radicals 79,80; an excess
of oxidation can exacerbate this issue. Free radicals can damage DNA
and can result in the alteration of a given gene.81 Iron is involved
in the production of DNA.82
The alteration of the iron state of the
blood can therefore also jeopardize the genetic integrity of the
cell.
What we see, therefore is that any alteration or interference of
iron metabolism in the body leads to serious and systemic degradation
in human health and functioning. In addition, the very mechanisms of
damage (as defined from a pathological perspective) to the cells are
identified as being factors of the Morgellons situation and they
fully satisfy the definition of a diseased organism.
It is this
comprehensive and systemic effect upon the body which necessitates
the call to integrative and holistic medicine with a strong
foundation in biochemistry.
It is not anticipated at this point that
a myopic perspective on either symptoms or effects is likely to be
beneficial at the level that is required to establish health.
15.
Identification of physiological conditions that are in probable
conjunction with the condition
Based upon the understanding that has been presented thus far, there
exists a set of physiological conditions that is expected to be more
likely to occur in the "Morgellons affected individual" than in the
general population.
It is a probabilistic offering only.
This
information is not intended to be diagnostic in any sense and the
postulates are presented solely as a result of analytic and
observational research. The information is offered to the medical
community for their evaluation and assessment as the issue is
approached with greater seriousness in the future.
There is no
guarantee or implied guarantee that any of the following symptoms or
conditions will occur; only that they may deserve consideration by
the medical community as the situation is researched further.
The
list of candidate effects upon the body may or may not include:
-
An increased level of acidity in the body (may be most easily
assessed by urine pH testing).
-
Diminished oxygen carrying capacity of the blood.
-
Lower energy levels due to interference in the ATP production
cycle; greater fatigue.
-
The presence of filament structures (ferric iron - anthocyanin
complexes) within oral samples.
-
Recent research indicates that the urinary tract may be equally
affected with the presence of the filament structures.
-
The presence of a bacterial-like component (chlamydia-like)
within or surrounding the red blood cells.
-
Chronic decreased body temperature.
-
Respiratory problems, including proclivities toward a chronic
cough or walking pneumonia-like symptoms.
-
Skin manifestation at the more developed levels (the skin is an
excretory organ).
-
The impact of increased oxidation, greater free radical presence
and their damaging effects upon the body.
-
Tooth decay or loss.
-
The smoking population may exhibit an increased incidence of the
condition due to additional oxygen inhibition within the blood.
-
Liver toxicity, gall bladder and bile duct complications.
-
Potential reduction in arterial transport; increased blood
pressure.
-
Potential proclivity toward increased cancer incidence due to an
expected increase in anaerobic metabolism.
-
Additional unidentified systemic damage in conjunction with the
pathological mechanisms of cell injury identified.
16. A Proposed
Spectral Analysis Project
A relatively simple method to assess whether or not the oxygen
content of the blood is abnormally low has been created.
The method
uses the combination of an ordinary computer scanner along with
statistical analysis. Before this method is outlined further, I
would like to give due credit to Fathima Shihana, BSc with the
authored paper entitled "A Simple Quantitative Bedside Test to
Determine Methemoglobin" from the Annals of Emergence Medicine.
83
This paper has served as the inspiration for the approach described
here.
A spectrometer is a relatively costly instrument and it availability
is limited. The paper above describes a method whereby an ordinary
scanner can be used to establish a calibrated relationship between
the color of blood (as recorded by a color scanner) and the loss of
oxygen content (methoglobinemia) within that same blood.
The
cleverness of the idea resides in the fact that a color scanner,
along with suitable analysis software, is essentially a spectrometer
in its own right. Any color combination may be broken down into
quantitative measurements of the red, blue and green channels of
that color, and a scanner ingeniously serves as a readily acceptable
spectrometer in its own right.
The paper referred to deals with situations of methoglobinemial that
be lethal or extremely injurious to life; the project here is
operating on a much more subtle level in an effort to determine both
lesser magnitudes of the condition (i.e., asymptomatic) and finer
gradations within.
Without the advantage of calibration against
known lab standards, the scanner still serves as an excellent and
simple tool for relative changes in the condition of the blood.
Highly oxygenated blood is a rich red in color. Oxygen deprived
blood is more bluish and color and blood devoid of oxygen is brown.
Our goal with the current project is to be able to determine
relatively minor (but nevertheless significant) shifts in the color
of blood from red toward the blue portion of the spectrum.
As shown below, a modern computer color scanner can be used as a
three channel (red, green, blue) spectrometer and it can be used to
establish a highly unique signature for an appropriate sample.
The
proposed project of blood spectral analysis processes the sample
data in a unique fashion, but the spirit of the research paper
referred to above remains the foundation of the approach.
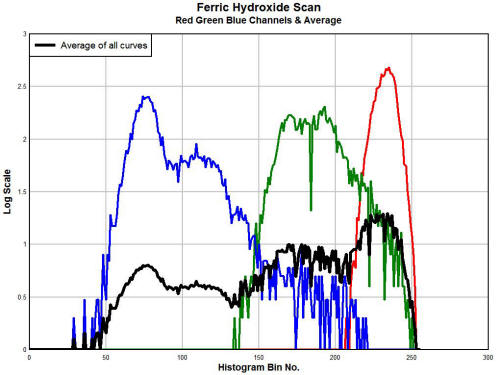
In practice, it has been found that the red channel is sufficient to
identify color shifts within the blood that indicate a decreased
oxygen supply to the blood.
If you would like to participate in this
research project, please send correspondence to info@carnicominstitute.org
and the particulars can be described. The only essential requirement
to participate in the project is that of a color scanner.
Please be
aware that no individual feedback or assessment will be provided to
those that participate in this project; any data will be handled in
a statistical sense and any data analysis will be presented to the
public in an anonymous fashion. If the medical community becomes
involved in this research the prospects of discussion may be able to
widen.
What follows below is an example of the processing that the project
entails, including the scan of a drop of blood by two separate
individuals and the statistical processing of a group of individuals
that have contributed to the research project:
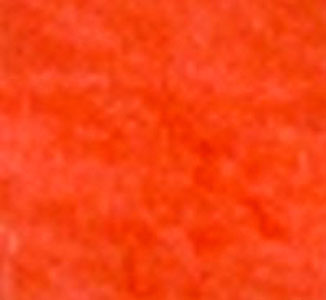
Individual A. A scan of the blood of the individual. This individual has no
outward manifestations of the "Morgellons" symptoms. The red channel
of the spectrum has been analyzed from a statistical perspective.
The individual has a relative rank in the +86% (-100% to +100%)
percentile, indicating a shift of the color toward the red portion
of the spectrum. This dominance of the red portion of the spectrum
indicates more highly oxygenated blood within the group sample.
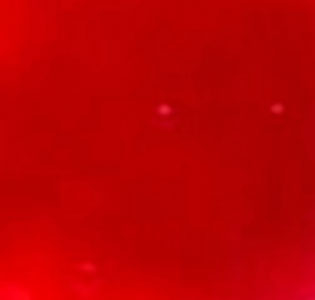
Individual B. A scan of the blood of the individual.
This individual has stated
and demonstrated significant skin manifestations of the "Morgellons"
symptoms. The red channel of the spectrum has been analyzed from a
statistical perspective. The individual has a relative rank in the
-92% percentile (-100% to 100%), indicating a shift of the color
toward the blue portion of the spectrum. This shift towards the blue
portion of the spectrum indicates a decrease in the oxygen level of
the blood of the individual.
This finding is in accordance with the
primary thesis of this paper.
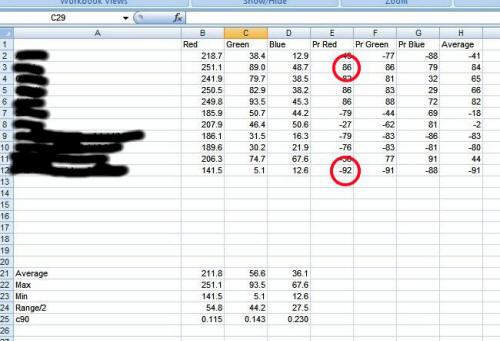
The spreadsheet analysis for the sample group.
The worksheet
analyzes the statistical properties of the sample group (11
individuals) with respect to the average spectrums of the red, green
and blue channels. In practice, it is found that the red channel
shifts in the spectrum are sufficient to characterize the deviations
in color. The color changes, or shifts, are an expression of the
oxygen content of the blood.
The sample group at this time is
limited and also has a high probability of being polarized with a
limited data set.
A broader sample group is expected to reveal a
more even distribution of oxygen supply and deficiency. If you would
like to contribute to this research project, please contact info@carnicominstitute.org
for the particulars.
No individual data will be provided to
participants; all analysis and presentation will be from an
anonymous statistical perspective.
17. A Review
of Proposed Mitigation Strategies
With the understanding of how a malady affects the body, we are in a
stronger position to develop strategies to mitigate the damage.
The
better approach is to put a stop to the problem, but that requires a
broader coordination and alliance than has been achieved thus far.
We can at least consider and establish some defenses while the
forces of political and social organization continue to arm
themselves.
What follows here are merely suggestions to consider;
they are in no way to interpreted as therapeutic or diagnostic in
approach. Each of the following strategies has been developed as a
direct response to laboratory conditions or academic study; they are
not formulated within any formal medical framework.
Each individual
is responsible for consulting with the medical professionals of
their choice and the following information is provided solely for
consideration within that consultation. Many of these items have
been mentioned previously and the list has accumulated in a gradual
fashion. Understanding the extent of the problem, it is not intended
that the "list" is complete; in fact it would seem that it is only a
beginning.
It will be noticed that many of these strategies can
apply to human health in general. The primary mechanisms of many
diseases are actually few in number and these have been enumerated
in the discussion of pathology above.
All being said, let us proceed with some strategies for mitigation
of the "condition".
-
Alkalization of the body would appear to be a beneficial practice
in general with respect to disease84,85,86. It has been identified
that the organism flourishes within an acidic environment.87,88
It
is also known that biochemical processes usually take place within a
specific pH range, including the growth of pathogenic forms.89,90,91
-
The research indicates that excessive oxidation is detrimental to
health. This topic has also been discussed previously in an earlier
paper.92
Common oxidizers include the bleaches, peroxides and ozone.
The research indicates, from the vantage point of this researcher,
that internal use of these substances is likely to be harmful to
human health. We do not solve the problem of oxidation within the
body by necessarily increasing the intake of oxygen.
Indeed, one of
primary arguments of this paper is that the blood of the affected
individual has been oxidized in a fashion that has the net effect of
decreasing the oxygen carrying capacity of the blood. Excessive and
misplaced oxidation also creates free radicals, which as been noted,
"wreak havoc in the living system."
We do not solve that problem by
taking more oxygen; we work on the problem by hindering the
oxidative process. The manner in which this process is conducted in
the chemical world is known as reduction. In common terms, the
appropriate term is that of an anti-oxidant, and many of us are
familiar with that parlance.
I take stock in the following statement, again from Coltrane:93
"Once free radicals are formed, how does the body get rid of them?
There are several systems that contribute to termination or
inactivation of free radical reactions:
1. ... Antioxidants
(e.g., vitamins, glutathion, transferrin..)
2. Enzymes."
The statements here are direct and understandable and come from a
standard textbook in pathology.
It is relatively straightforward
that if a problem of excessive oxidation exists within the body, one
should strongly consider the role that anti-oxidants play in
reversing those effects. It is equally inadvisable, from this
researcher's point of view, to compound the issue with the addition
of known strong oxidizers internal to the body
Vitamins, across the board (A, B, C, D, E) are powerful antioxidants.
An additional powerful antioxidant identified in the research is
that of glutathion. The role of Vitamin C (ascorbic acid) in the
inhibition of the culture growth has already been described. There
remain many additional anti-oxidants of importance in human
health.94
-
Increasing the utilization and absorption of existing iron within
the body. Iron is certainly one of the most important elements of
the body. Referring to the Linus Pauling Institute,95
"Iron has the longest and best described history among all the
micronutrients. It is a key element in the metabolism of almost all
living organisms. In humans, iron is an essential component of
hundreds of proteins and enzymes."
One of the findings from the study of coordination chemistry
described above is that iron has the ability to bond with numerous
other molecules. For example, iron (in the Fe2+ state)
preferentially bonds to oxygen. If the iron is altered to the Fe(3+)
state. it will no longer bond to oxygen. In this modified state, the
iron will then form additional bonds to other molecules, many of
which are harmful as has also been described above.
The idea of a chelator is to keep the oxygen bound in a protected state where it
can not bind so easily with other, often harmful, molecules. Heme
itself, within hemoglobin, is a classic example of a chelator. If
our iron has been altered to where it becomes free or bound to other
molecules (potentially harmful ligands), the solution to that
problem would not seem to be to take more iron, any more than
increasing the oxygen intake is expected to resolve a problem of
oxidation.
The more effective solution would appear to be to keep the iron in a
chelated state, where it is bound and protected by the expected
molecules and proteins such as heme in the body.
This therefore
suggests that increased attention would be devoted to the study and
role of chelators in human health. It does not seem reasonable that
we would automatically pursue a path of increasing iron intake;
indeed this process can be quite harmful and dangerous to human
health.
Again, the importance of consultation with the medical
professionals of choice is unequivocally stated; the stakes of the
issues we are speaking of are of the highest importance.
-
The inhibition of the growth of iron-consuming bacteria (and
bacteria-archea like) forms.
We know now that the organism uses iron for its existence and
growth. It appears that iron in the further oxidized state (i.e.,
Fe3+) is of primary benefit to the organism. We also know, in
retrospect, that iron is a critical metabolic element within many of
the bacteria (or bacteria-archaea like forms).
One strategy that
develops with such organism is that of inhibiting the ability of the
organism to access or metabolize the iron. This once again brings up
the idea of a chelator. This topic has also been discussed in an
earlier paper, and introduced the role of human breast milk and its
resistance to bacterial forms in infant growth.96
Lactoferrin (found
in whey) was identified as a potential strong chelating protein
within that research. Transferrin is another protein chelator within
the human digestive tract that serves a similar purpose, i.e.,
binding of the iron and consequently it becomes less accessible to
iron-consuming bacteria (or bacteria-archea like forms).
-
Improving the flow of bile in the system to further alkalize the
body and aid the digestive system. The liver, the gall bladder and
the bile duct play an extremely important role in alkalizing the
digestive tract. For those that demonstrate a persistent acidic
condition within the body it may be beneficial to learn of the
importance of bile production and its alkalizing function.
An
excellent introduction to the physiology of this important aspect of
human health may be found at the following site:
Video Series:
Liver, Gall Bladder and Bile Duct Physiology.
An acidic condition can easily be created with a blockage of the
bile duct, as the bile is the alkalizing agent within the intestine.
Gall bladder removal and gall stones appear to be a frequent
occurrence; this would suggest that overloads of toxicity to the
liver could well be at the root of this problem.
Non-invasive
methods of breaking down gall stones (conglomeration of bile) are
available to consider, such as Chanca Piedra (breakstone). If the
bile flow is restricted, an acidic condition within the body is
expected to exist. Knowledge of the physiology of the liver, gall
bladder, bile duct and its relationship to digestion may be
beneficial in mitigating the consequences of acidity within the body
and digestive system.
-
Detoxification of the liver (toxin removal and the breakdown of lipids (fats)). One of the many functions of the liver is to break
down fats with the use of bile. If the bile is not being produced or
flowing within the digestive system, the fats will accumulate within
the liver. The liver also removes toxins from the body. If the liver
is not functioning correctly (e.g., from an accumulation of fats or
the lack of bile flow) serious consequences to health will ensue.
As an aside and as an unreported event, I received information
indirectly many years ago from a U.S. Naval pathologist. This
pathologist was provided certain microphotographs of blood samples
that I had taken. The research was not mature to the point that it
is now, however, it was mentioned by the pathologist that the
condition I was reporting is indeed commonly being observed.
This
pathologist, to the best of my recollection, attributed the source
of the problem to the failure of a particular enzyme within the
liver. At this point I cannot recall the name of the specific
enzyme. It is nevertheless of great interest to understand that the
liver now exists as one of the primary targets of systematic failure
within the Morgellons research that is underway.
There are many serious consequences to a liver that is overloaded
with toxins. Another example of damage, beyond fatty accumulation,
is what is called lipid peroxidation. Lipid peroxidation is caused
by the presence of free radicals and it involves the deterioration
of fats through an oxidation process. In layman terms, the situation
can be equated to that of rancid, or spoiling fats.
The value of knowledge on detoxification of the liver now becomes
apparent. The free flow of bile (indicated by peristalsis, or
rhythmic contractions of the intestine) may be one of the first
conditions to indicate improved digestive activity. Liver
detoxification is an important subject in its own right and is
likely worthy of serious investigation, study and application by
each of us.
The purpose here is to indicate simply another aspect of
human health that is deeply enmeshed in the path to better health,
and that is a smoothly functioning liver.
-
Enzymes. What we are learning here is that the road to better
health and the prevention of disease, regardless of the source,
requires an integrative process. It may require more effort than
many of us are willing to expend. We soon become aware, especially
when we seek answers to the serious problems posed above, that we
must start to learn how the body actually works.
We must start to
learn the relationships of one part of the system to another. It is
a fascinating and hopefully beneficial process if you are willing to
pursue that pathway, but it will not be accomplished without effort
on your part. It requires the same of me.
Another simple example of another important relationship is the
following. Essentially every chemical reaction that takes place in
the body requires the use of enzymes. With an understanding and
appreciation of this profound statement, my appreciation for
understanding the nature and role of enzymes is now earnest.
Enzymes
are actually an amazing chemical phenomena; they essentially cause
something to happen that would not happen otherwise, and the enzymes
themselves are not even changed in the process. They provide an
alternative energy pathway to get something done, and with the
overall reaction requiring less energy in the process.
One analogy
given is that of a tunnel through a mountain; you can either climb
over the mountain and expend a great deal of energy and effort (and
maybe never make it over the top) or you can go through a tunnel if
one happens to be there. An enzyme is somewhat analogous to the
tunnel though the mountain.
An example of an enzymatic, or catalytic, reaction, is shown in the
video segment within this report and above. In this case, hydrogen
peroxide is added to iron (ferric) oxide. What the observer sees is
a vigorous bubbling reaction. What is occurring in the reaction is
that the hydrogen peroxide is being decomposed, or broken down into
oxygen and water.
The iron in the reaction serves as the catalyst.
If you study this reaction long enough, you will find the iron oxide
is not changed no matter how long you watch it. It is
counter-intuitive, as when we see vigorous bubbles reacting to iron,
we expect the iron to visibly change or deteriorate in the process.
It does not.
But the reaction would not occur without the iron
present.
[Edit : Dec 01 2011 : A drop of one or two degrees in body temperature can have a marked
effect on body metabolism and enzyme activity. It is expected that
this level of decrease in body temperature could correspondingly
decrease enzyme activity on the order of 10% to even 40%97,98.
As we
have learned of the one to one correspondence between metabolism and
enzyme activity, major impairment of our metabolism and functioning
is expected with decreased body temperatures.
There is an
accumulated body of information that indicates that the body
temperatures of the general population may now well be lower by this
same amount of one to two degrees. This topic exists as a focal
point of future research.]
Now that we see more clearly the importance and function of enzymes,
we can also understand why a lacking enzyme within the liver might
be very serious business. It therefore behooves us to add an
additional field of study to our pursuits in biochemistry and health
restoration, and this is the study of enzymes.
We must learn what
enzymes are likely to be involved within the systems that are known
to be failing (circulation, respiration, digestion, etc) and what
can be done to restore the deficiencies. Once again, I can see no
alternative to wholistic and integrative medicine and health
research in the solution to the problems before us.
In summary, I now see five major challenges before us with the "Morgellons"
issue based upon the research that I have conducted to date:
-
The iron within the blood, to a partial degree, is being changed
in a way that it no longer binds with oxygen at the normal levels
that are expected. This same iron is being used by the organism to
sustain its own existence and growth. Diminished oxygen carrying
capacity of the blood is therefore expected to occur in coincidence
with the severity of the condition.
-
The presence of free radicals are likely to increase in number
and extent as a result of the oxidation process mentioned
immediately above. Free radicals are known to "wreak havoc in the
living system", as has been mentioned earlier.
-
The altered iron (Fe3+
vs. Fe2+) now binds to other molecules,
many of them toxic or harmful to health, instead of oxygen as is
expected. Several of these alternative ligands are known respiratory
inhibitors, and therefore further exacerbate the failures in
respiration.
-
The bacteria-like form, which appears to be at the origin of the
pathogen, itself binds to oxygen to support its own existence. This
is in addition to the consumption of iron already identified. This
combination further increases the severity of consequence to human
health.
-
The presence of the organism, as encountered, appears to be
extensive within the body. It appears to occur within the
circulatory, digestive and urinary systems as a minimum.
A few, and only a few, suggestions have been given about how these
problems can be approached.
These strategies are by no means
intended to encompass all needs before us. The will hopefully,
however, provide a stepping stone to the further research that
exists before us. These problems will never be solved with ignorance
or apathy.
I encourage you to participate in the process of
resolution and accountability, and to support those who act on that
same behalf.
Note: I am not
offering any medical advice or diagnosis with the presentation of
this information. I am acting solely as an independent researcher
providing the results of extended observation and analysis of
unusual biological conditions that are evident.
Each individual must
work with their own health professional to establish any appropriate
course of action and any health related comments in this paper are
solely for informational purposes and they are from my own
perspective.
References
1.
Free Radicals in Biology and Medicine, Dr. P.K. Joseph,
PhysicianByte.com
2.
Hemoglobin,
Wikepedia, July 2011.
3.
Hemoglobin, Chemistry Explained, N.M. Senozan.
4.
Iron in Cell Culture, Sigma-Aldrich, sigmaaldrich.com.
5. Biochemstry Demystified, Sharon
Walker, PhD, 2008, McGraw Hill, p. 264.
6.
Iron, University of North Carolina at Pembroke.
7.
Determination of Iron with 1,10-Phenanthroline, University
of Tennessee, Knoxville, Department of Chemistry.
8.
Morgellons : A
Discovery and a Proposal, C.E. Carnicom, February 2011.
9. Ibid., Carnicom.
10.
IUPAC Gold Book
- Fenton Reaction, IUPAC Compendium of Chemical Terminology.
11.
Qualitative Analysis Tests,
Chemical Identification
Tests for Positive Ions, Phil Brown, PhD.
12.
Qualitative Analysis Tests,
Chemical Identification
Tests for Negative Ions, Phil Brown, PhD.13. Easy
Chemistry, Josehp A. Mascetta, M.S., 2009, Barron's Educational
Series, pp. 373-375.
13.
Definition of Coordinate Covalent Bond, Everything Bio, An
All Encompassing Bio Resource.
14. Oxford Dictionary of Chemistry,
2000, Oxford University Press, p 120.
15. A-Z Chemistry, Andrew Hunt,
2003, by McGraw-Hill, p. 101-102.
16.
Coordinate Covalent Bond - Definition, wordiq.com.
17. Modern Biology, Albert Towle,
1999 by Holt, Rinehart & Winston. pp.939, 1108.
18. Biology, Neil A Campbell, PhD.
1993 by Benjamin Cummings Publishing Co. p. 843.
19. Campbell, p 844.
20.
Morgellons : A
Discovery and a Proposal, Carnicom.
21. Free
Radicals in Biology and Medicine, Dr. P.K. Joseph.
22. Ibid., Joseph.
23.
Morgellons, A
Fourth Match, Carnicom, 2008.
24.
Morgellons: The
Wine-Peroxide Test, Carnicom, 2008.
25.
Morgellons: The
Extent of the Problem, Carnicom 2010.
26.
Morgellons: A
Status Report, Carnicom, 2009.
27. Chemistry Made Simple, John T.
Moore, Ed. D., 2004, Broadway Books, p 134-135.
28. Foundations of College
Chemistry, Morris Hein, 1996 by Brooks/Cole Publishing Co., p
157-158.
29.
American Druggist, Volume 22, Google Books, p 197.
30.
Oxidation of Ascorbic Acid, Chemistry Comes Alive, Volume 5.
31.
Growing Metal Crystals; Electrolysis of Metal Salts,
Derek's Mundane Web.
32.
Electroplating, Electrochemistry Encyclopedia, Case Western
Reserve University, Ernest B. Yeager Center for Electrochemical
Sciences.
33. General Chemistry, Linus
Pauling, Dover Publishing, 1988, pp. 512-520.
34. Can
Iron Ore Be Extracted by Electrolyis?, Yahoo Answers,
Malaysia.
35.
Iron (III) Oxide, Journal of Chemical Education, Vol 78, No.
10, October 2001.
36.
Catalytic Decomposition of Hydrogen Peroxide and 2-chlorophenol
with iron oxides, Water Research, Vol. 35, Issue 9, June
2001, pp.2291-2299. Abstract.
37.
Ligand,
Wikipedia.
38.
Thinkwell Chemistry, Transition Elements, Dr. Dean Harmon
and Dr. Gordon Yee.
39.
Spectrochemical Series, ChemWiki, University of California
at Davis.
40. Ibid.,
Ligand,
Wikipedia.
41.
Transition Metals and Coordination Chemistry, Chapter 20
Notes, University of Washington, p.5.
42. Ibid, Walker., Chemicals That
Impede Hemoglobin Functions - Poisons. P 272
43. Ibid., Thinkwell Chemistry.
44.
Altered Blood, Carnicom, Jun 2011.
45.
Definition of Methemoglobinemia, MedicineNet.com.
46.
Causes and Clinical Significance of Increased Methemoglobin,
Asociacion Espanola de Farmaceuticos Analistas.,
www.aefa.es.
47. Ibid., AEFA.
48. Ibid., AEFA.
49. Ibid., Walker, p. 27.
49.
Respiration, Royal Society of Chemistry, www.rsc.org.
50.
Energy
Changes in Chemical Reactions, Avogadro Web Site,
www.avogadro.co.uk.
51. Ionization Potentials of Atoms
and Atomic Ions, Handbook of Chemistry and Physics, 82nd
Edition, CRC Press, p 10-175.
52.
Properties of Atoms, Radicals and Ions, Table 4.11
Bond Dissociation Energies, Department of Inorganic
Chemistry, University of Buenos Aires.
53. Chemistry, The Central Science,
Theodore L. Brown, PhD, 2006 by Pearson Education - Prentice
Hall, p. 1036.
54.
Morgellons: A New Classification. Carnicom. Feb. 2010.
55.
How Some Bacteria May Steal from their Human Hosts, Science
Daily, Aug 2008.
56.
Do Bacteria Affect the Rusting of Iron?, Douglas Bintzler,
www.ehow.com.
57.
Iron Bacteria,BioVir
Laboratories, Inc., www. biovir.com.
58.
Bacteriology - Chapter Three - Nutrition, Growth and Energy
Metabolism, Dr. Alvin Fox, Microbiology and Immunology
On-line, University of South Carolina School of Medicine.
59.
Siderophore Uptake in Bacteria and the Battle for Iron with the
Host; A Bird's Eye View, Chu BC, Biometals, Aug 23. 2010.,
Abstract.
60.
Iron Metabolism in Pathogenic Bacteria, Colin Ratledge,
Annual Review of Microbiology, Vol 54. p. 881-941, Abstract.
61.
Iron Metabolism Bacteria, Ken Burnside,
www.ehow.com.
62.
Microorganisms Pumping Iron; Anaerobic Microbial Iron Oxidation
and Reduction, Karrie A Weber, Nature Reviews Microbiology,
Oct. 2006, Abstract.
63.
Free Iron
in Bacteria, Jim Imlay PhD, Department of Microbiology,
University of Illinois, Urbana-Champaing, Society for Radical
Biology and Medicine.
64.
Topic 6, Coordination Compounds, Georgia Tech University,
Chemistry and Biochemistry, www.chemistry.gatech.edu.
65. Enterobactin,
Wikipedia.
66.
Siderophore Electrochemistry: Relation to Intracellular Iron
Release Mechanism, Proceedings National Academy of Science,
Vol. 75, No 8, pp. 3551-3554. Aug 1978, Chemistry.
67.
Carnicom Institute,
www.carnicominstitute.org.
68.
A Spectrofluorimetric Sensor Based on Grape Skin Tissue for
Determination of Iron(III), Minghui Zhang, Bulletin Chemical
Society of Ethiopia 2010 24(1), 31-37.
69.
The Role of Iron and Tion in Discoloration of Berry and Red Beet
Juices, Heikki Pyysalo, Zeitschrift Fur
Lebensmitteluntersuchung Und - Forschung A, Volume 153, Number
4, 224-233. Abstract.
70.
Blue Metal Complex Pigments Involved in Blue Flower Color,
Kosaku Takeda, Proceedings of the Japan Academy, Series B,
Physical and Biological Sciences, Vol 82 (2006), No. 4, p
142-154. Abstract.
71.
Determination of Anthocyanins in Red Wine Using a Newly
Developed Method Based on Fourier Transform Infrared
Spectroscopy, A. Soriano, Food Chemistry, Vol. 104, Issue 3,
2007, P1295-1303. Abstract.
72.
Iron-Polyphenol Complex Formation and Skin Discoloration in
Peaches and Nectarines, Guiwen Cheng, Journal of the
American Society for Horticultural Science, Vol 122, Jan. 1997,
p. 95-99.
73. Robbins Pathologic Basis of
Disease, Ramzi S. Cotran, M.D., 1989, W. B. Saunders Company,
4th Edition
74. Ibid., Cotran, p 3.
75.
A
Mechanism of Blood Damage, C.E. Carnicom, Dec. 2009.
76.
Enzymes, Cliffs Notes,
www.cliffsnotes.com
77. Ibid., Walker. p 185.
78. Micronutrient
Information Center, Linus Pauling Institute., Oregon State
University.
79. Ibid., Johnson.
80. Antioxidants, Better Health
Channel, Victorian (Australia) State Government.
81. Ibid., Walker, p 169.
82. Ibid., Linus Pauling Institute.
83. A Simple Quantitative Bedside
Test to Determine Methemoglobin, Fathima Shihana, BSc, South
Asian Clinical Toxicology Research Collaboration, Annals of
Emergency Medicine, Vol. 55, No 2. Feb 2010.
84.
Acidity,
Disease and Cancer, Health News,
http://www.healthnews-nz.com.
85. Ibid., Cotran, p. 4.
86.
Body Acidity, Disease Prevention and More About Aspartame,
Stephen Sampson, www.associatedcontent.com.
87.
Morgellons: A Discovery and A Proposal, C.E. Carnicom, Jun.
2011.
88.
Morgellons: In the Laboratory, C.E. Carnicom, Jun. 2011.
89. Biochemistry, Philip Kuchel,
PhD, (2009, McGraw-Hill, 32).
90. Biochemistry for Dummies, John
Moore, EdD, (2008, Wiley Publishing, 29).
91. Brown, Steven; Chemistry 102a
Laboratory Manual, Kendall Hunt Publishing Company, 1996., p
125.
92. Ibid., A Discovery and A
Proposal, C.E. Carnicom.
93. Ibid., Cotran, p. 12.
94. Ibid., A Discovery and A
Proposal, C.E. Carnicom.
95. Ibid., Linus Pauling Institute.
96. Ibid.,
Morgellons: In the Laboratory, C.E. Carnicom.
97.
Temperature Effects - Introduction to Enzymes, Worthington
Biochemical Corporation, www. worthington-biochem.com
98. The
Cold Body Page, http://www.mall-net.com/mcs/coldbody.html.
|