Geochemical Overview of the Lake
Berry Lyons
Department of Geology,
University of Alabama, Box 870338, Tuscaloosa AL 35487-0338,
U.S.A.
p (205) 348-0583, f (205) 348-0818,
blyons@wgs.geo.ua.edu
Lyons discussed how the major ion chemistry of the lake might
have evolved based on the French research on the chemistry of
the Vostok ice core. Because the hydrogen ion is a major caption
in the ice during interglacial times, the lake’s water could be
acidic.
This might lead to enhanced leaching
of particulate matter within or at the sediment-water interface
of the lake. In addition, he described the possible N:P ratios
of the water (again, based on the ice core results), and
suggested that the lake could be very P deficient.
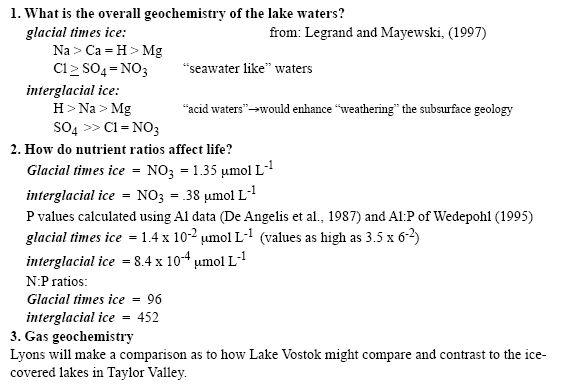
Technologies for Access Holes and
Thermal Probes
Stephen R. Platt
Snow & Ice Research
Group (SIRG), Polar Ice Coring Office, Snow & Ice Research
Group,
University of Nebraska-Lincoln, 2255 W Street, Suite 101,
Lincoln, NE 68583-0850, U.S.A.,
p (402) 472-9833, f (402) 472-9832,
srp@unl.edu
The Snow & Ice Research Group (SIRG) at the University of
Nebraska-Lincoln has conducted a comprehensive analysis of the
technological challenges associated with delivering a
cryobot-hydrobot transporter vehicle to the surface of Lake
Vostok, and has developed a plan that we believe has the highest
chance of success and lowest cost consistent with logistical,
technical, and time constraints.
The proposed course of action uses a
hot water drill to produce a 50 cm diameter access hole
approximately 3700 m deep. An instrument carrying thermal probe
(the cryobot) will then be deployed from the bottom of this hole
to penetrate the final few hundred meters of ice and deliver a
hydrobot exploration vehicle to the surface of the Lake.
A division of SIRG, the Polar Ice Coring Office, has a proven
capability for drilling 2400 meter deep access holes in ice
using a hot water drilling system at the South Pole. The current
drill design can be modified to achieve depths of 3500-3700 m.
Hot water drilling will not produce a permanent access hole
because the hole will begin to refreeze as soon as the water
stops circulating.
Once the access hole freezes over,
the lake would remain sealed from the outside world, even as the
probe entered it. However, because the drilling fluid for this
technique is water, the risk of contaminating the Lake is
greatly reduced compared to alternative drilling techniques.
Furthermore, this is the fastest method for producing large,
deep access holes in the ice.
Once the drill equipment is
assembled on-site, 3700 m deep holes can be drilled in less than
two weeks SIRG has also developed thermal probes for making
in-situ measurements of the properties of the Greenland and
Antarctic ice sheets. A thermal probe is an instrumented
cylindrical vehicle that melts its way vertically down through
an ice sheet.
At Lake Vostok, a thermal probe
would be lowered to the bottom of the access hole created by the
hot water drill, where it would start its descent in to the
lake. The probe can be configured to house instruments which
measure parameters indirectly through windows in the outer wall
of the vehicle, or directly by using melt-water passed through
the probe.
This approach is fundamentally
different from other means of sampling the physical parameters
of ice sheets which usually rely on recovering ice cores. A
cable housed within the upper section of the probe unwinds as it
moves down through the ice. This cable is used for both data and
electrical power transmission between the probe and the support
equipment on the surface of the ice sheet.
The probe can only make a one-way
trip down through the ice because the melt-water re-freezes
behind the probe so it is not recoverable.
SIRG is currently
doing the preliminary design work for modifying existing probes
for use as instrument delivery vehicles, and for integrating
in-situ measurement techniques for physical, chemical, and
biological phenomena with the cryobot-hydrobot delivery
platform.
Helium isotopic measurements of
Lake Vostok
Brent D. Turrin
Lamont-Doherty Earth
Observatory, Columbia University, Route 9WPalisades New York,
10964, U.S.A.
p (914) 365-8454, f (914) 365-8155,
bturrin@ldeo.columbia.edu
Helium isotopic measurements will help provide information on
the tectonic environment of Lake Vostok.
The input of He into
Lake Vostok will come from three discrete sources, atmospheric,
crustal, and mantle. These sources of He have distinctly
different isotopic signatures. Atmospheric He, accounting for
the decay of natural tritium to 3He, will have a R/Ra between 1
to 1.5. Atmospheric He enters the lake via melting ice at the
ice-water interface.
If Lake Vostok is located on old stable continental crust, the
measured He (helium) will have a R/Ra of 0.01. Because crustal He is
dominated by a large input of 4He from radioactive decay of U
and Th. On the other hand, if Lake Vostok is located in an
active rift environment, the flux of mantle He (R/Ra=6) into the
lake would increase the measured He R/Ra to values significantly
greater than one.
The He sampling protocol must sample a profile thorough the
water column. This is necessary to determine the mixing
structures between different He sources.
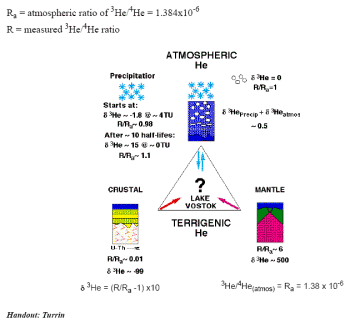
Molecular Characterization of
Microbial Communities
José R. de la Torre
& Norman R. Pace
Department of Plant and
Microbial Biology, University of California Berkeley, Berkeley,
CA 94720-3102 U.S.A.
p (510) 643-2572, f (510) 642-4995,
jtorre@nature.berkeley.edu
It has recently become accepted that microbial organisms thrive
in habitats previously deemed too extreme to support life.
Lake Vostok represents a new and unexplored habitat, subglacial
lakes, which may contain untold biodiversity despite the
challenges presented by the physical environment: extreme
pressure, darkness, cold and presumably few available nutrients.
The use of molecular techniques in
studying microbial populations presents several advantages over
traditional survey methods. Most importantly, these methods
eliminate the need for laboratory cultivation, since the vast
majority (>99%) of microorganisms are refractory to laboratory
cultivation using standard techniques. This molecular approach
is based on the use of ribosomal RNA (rRNA) sequences to
identify population constituents, and to deduce phylogenetic
relationships.
This sequence information is
obtained by either directly cloning environmental DNA, or by
cloning amplified polymerase chain reaction (PCR) products
generated using oligonucleotide primers complimentary to either
universally conserved or phylogenetic group specific sequences
in the rDNA.
Comparison of these cloned sequences with those of
known rRNA genes reveals quantifiable phylogenetic
relationships, independent of morphological and physiological
variations, between constituents of the studied community and
previously characterized organisms.
These data allow the inference of
physiological and metabolic properties based on the properties
of known relatives within particular phylogenetic groups.
This
sequence information can also be used to design
fluorescently-labeled oligonucleotide probes to examine the
morphology and physical distribution of the novel organisms in
the environmental setting.
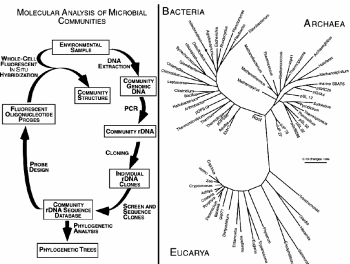
Contributions of Ice Sheet Models
to Understanding Lake Vostok
Christina L Hulbe
Code 971, NASA Goddard
Space Flight Center, Greenbelt, MD 20771, U.S.A.,
p (301) 614-5911, f (301) 614-5644,
chulbe@ice.gsfc.nasa.gov
Dynamic/thermodynamic
numerical models of ice sheet flow should play a role in several
aspects in the exploration of Lake Vostok.
First, models can be
used to characterize the present-day physical environment of the
lake. For example, by providing a 3-dimensional view of ice
temperature and age, estimating the influx of debris carried by
ice flow, and estimating the horizontal flux of ice sheet basal
melt-water into the lake.
When coupled with a numerical model
of lake water circulation, an ice sheet model can predict the
spatial pattern and rate of melting and freezing above the lake.
Second, numerical models can investigate the climate-cycle
history of the lake. Changes in ice sheet mass balance over the
time since the lake was isolated from the atmosphere are likely
to have affected Lake Vostok’s area extent, its sediment
content, and melt-water flux.
To perform such computations, ice
sheet models will need accurate, well-resolved basal topography
of the region around the lake and as much information about
basal geology and geothermal heat flux as possible. Other input
data, such as present-day surface elevation and the local
climate record, are available. Indeed, the closeness of the
Vostok ice core climate record is ideal.
Numerical-model studies of both
present and past lake environments would be useful in both
site-selection prior to direct contact with the lake and in
interpretation of data retrieved from lake exploration.
Implications of Ice Motion Over
Lake Vostok
Ron Kwok
Jet Propulsion
Laboratory, California Institute of Technology, 4800 Oak Grove
Dr.
Pasadena, CA 91109, U.S.A.
p (818) 354-5614, f (818) 393-3077,
ron.kwok@jpl.nasa.gov
Ice motion estimates show that the subglacial lake exerts
considerable control over the regional ice dynamics.
As the ice
flows pass the grounding line, there seems to be a pronounced
southward component of motion with a profile which increases
slowly at the northern tip of the lake and then rather rapidly
starting at approximately 100 km along the length of the lake.
Critical to the understanding of
past trajectories of the ice recently cored at Vostok Station,
and the interpretation of internal layers of the ice sheet from
radio echo sounding measurements, the characteristics of the ice
motion of the ice sheet as it flows over the lake are important.
If flow is normal to the contours over the center of the lake,
ice from the lower parts of the Vostok ice core spent on the
order of 100,000 yrs traveling down the length of the lake.
In this case, dating core layering
should be regular and accurate. If there was a westward
component, the age-depth relation in the previously grounded ice
core would be less regular than for transport down the lake. The
ice motion field also raises numerous interesting questions
concerning thermal and mechanical processes in the ice sheet.
It
will help in the modeling of bottom melt and accretion;
processes which might help localize areas where ecosystems could
most likely exist.
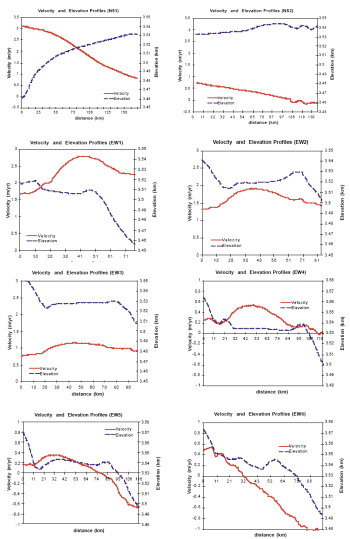
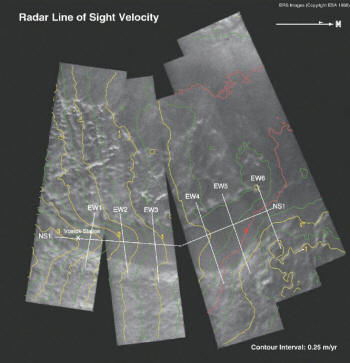
The Study & Evolution of an Ancient Ecosystem & Its Evolution
Todd Sowers
Penn State University, Geosciences Dept., 447 Deike Bldg.,
University Park, PA, 16802 U.S.A.,
p (814) 863-8093, Lab 863-2049 or 863-3819, f (814) 863-7823,
sowers@geosc.psu.edu
Why study the Lake?
One fascinating aspect of the lake involves the notion that we
may be able to study an ancient ecosystem that has evolved for
millions of years.
This ecosystem has been effectively isolated
from almost every aspect of the biosphere as we know it.
As
such, the organisms which inhabit the lake have adapted to a
very different environment compared to most of the near-surface
ecosystems studied to date. In my mind, the most important
reason to study the lake is to document the evolution of the
biota within the lake. The results will not only shed light on
evolutionary biology here on Earth, but it will also help in the
search for life on other (cold) planets.
In terms of my specific contribution to the study of Lake
Vostok, I’d be very interested in looking at the isotope
systematics of the lake. Specifically, I’d like to look into the
stable isotopic composition of O2, N2, and Ar clathrates which
are liable to be floating near the water/ice interface.
There
are two interesting aspects of such a study which will need to
be considered in parallel; 1) the possibility of dating the lake
and 2) providing some constraints on the biogeochemical cycling
of O2 and N2 within the lake.
1) The 18º/16º of O2 in the lake may provide some information
regarding the age of the lake.
To use the d18O of clathrate O2, we must first assume that the
d18O of paleoatmospheric O2 has followed the d18O of sea water
as it apparently has (to a first approximation) over the last
400,000 years (Bender et al., 1994; Jouzel et al., 1996; Sowers
et al., 1993).
Then, using the d18O of benthic forams covering
the Tertiary (Miller et al., 1987) as a proxy for the d18O of
sea water (and paleoatmospheric O2), we may be able to ascertain
the youngest age of the lake by analyzing the d18O of clathrate
O2 from the lake.
If the d18O of O2 is within 1‰ of the present
day value, then we can safely say that the lake is probably less
than 2.2 myr old. If, on the other hand, the d18O of O2 is
between 1 and 3‰ lower than today, then we can say that the clathrates (and lake) are probably between 2.2 and 50 ma (myr
before present).
Values which are lower than 3‰ could be
interpreted as signaling clathrates which are more than 50 myr
old.
2) By studying the stable isotope systematics of O2, N2, and Ar,
we may be able to learn something about the biogeochemical
cycling of these bioactive elements within the lake.
Assuming
organisms can be cultured and incubated under conditions
approaching Lake Vostok, (and the organisms use/produce O2 and
N2 as part of their metabolic activity), we can determine the
community isotope effect for these gases using laboratory
incubations.
Having this data in hand, along with the isotope
measurements on the air clathrates from the lake, we may be able
to provide some qualitative estimates of the longevity of the
ecosystem via simple isotope mass balance.
Modeling the thermal forcing of the circulation in Lake Vostok
David Michael Holland
Courant Institute of Mathematical Sciences, 251 Mercer Street,
Warren Weaver Hall, 907, New York University, MC 0711, New York
City, New York, 10012 U.S.A.
p (212) 998-3245, f (212) 995-4121,
holland@cims.nyu.edu
Lake Vostok is situated at the base of the huge Antarctic Ice
Sheet.
The isolation and remoteness of the lake imply that it
will have a circulation driven by the heat and freshwater fluxes
associated with phase changes at the ice sheet - lake surface
boundary.
While geothermal fluxes would also play a role at the
lake bed interface, the nature of these important, but poorly
known fluxes for Lake Vostok, are not considered in the present
discussion. A hierarchy of formulations that could be used to
describe the heat and mass transfer processes at the lake
surface are presented. The main difference between them is the
treatment of turbulent transfer within the lake surface boundary
layer.
The computed response to various levels of thermal
driving and turbulent agitation in the upper layers of the lake
is discussed, as is the effect of various treatments of the
conductive heat flux into the overlying ice sheet.
The
performance of the different formulations has been evaluated for
the analogous environment of an oceanic cavity found beneath an
ice shelf.
In an effort to understand what the physical
circulation is in the lake and subsequently of what relevance it
might be to chemical and biological activity in the lake, the
following investigation is proposed:
An investigation of the
details of the thermal interaction between the lake and the
overlying ice sheet could be pursued by building on existing
theoretical and modeling studies of other cold liminological/oceanographical
environments.
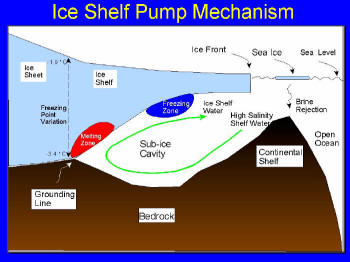
The detection of life - Nucleotide fingerprints
David M. Karl
School of Ocean and Earth Science and Technology, University of
Hawaii, Honolulu, HI 96822, U.S.A.
p (808) 956-8964, f (808) 956-5059,
dkarl@soest.hawaii.edu
Perhaps the first question that one should ask about “life” in
Lake Vostok is... is there any?
If the answer is yes, then one
needs to ask how much life is there, how rapidly is the crank
turning and what kind of life forms are present. Although there
are numerous methods available to address these fundamental
ecological questions, only a relatively few have the sensitivity
required for the detection of low standing stocks of
microorganisms that might occur in the hyperoligotrophic Lake
Vostok.
Adenosine 5’-triphosphate (ATP) is present in all living cells
where it functions as an essential link between energy
generation and biosynthesis and as a precursor for RNA and DNA
synthesis.
Furthermore, in concert with related cellular
nucleotides (e.g., ADP, AMP, GTP, cAMP, ppGpp), ATP also serves
to regulate and direct cellular metabolism. In addition, ATP and
associated nucleotide biomarkers can be extracted from cells and
measured in situ; hence sample return is not mandatory, although
it is desirable.
ATP has already proven to be useful in many ecological studies
of remote and extreme environments including the deepest
portions of the Aleutian Trench (>7500m), hydrothermal vents and
ice covered polar habitats.
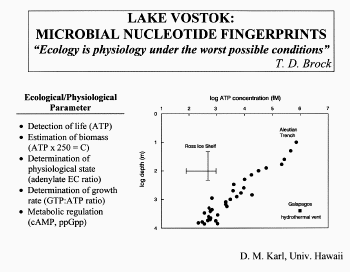
Alternative Mechanisms for Organic Syntheses and
the Origin of Life - Lake Vostok as a Case Study
Luann Becker
University of Hawaii, Manoa, 2525
Correa Rd., Honolulu, HI 96822, U.S.A.
p (808) 956-5010, f (808) 956-3188;
lbecker@soest.hawaii.edu
One of the more exciting new fields of research that is emerging
within the deep-sea drilling program is the search for a
subsurface biosphere.
This field has developed as a result of
the study of extreme environments and their possible link to the
first living organisms that inhabited the early Earth.
Recent experimental data show that amino acids can be activated
under plausible ‘Prebiotic’ geologic conditions [nickel, iron (Ni,Fe)
sulfide (S) and carbon monoxide (CO) in conjunction with
hydrogen sulfide (H2S) as a catalyst and condensation agent at
100oC, pH 7-10 under anaerobic, aqueous conditions; Huber and Wachtershauser (1998)].
These findings support a thermophilic
origin of life and the early appearance of peptides in the
evolution of a primordial metabolism.
Other research efforts have focused on identifying alternative
energy sources available in hydrothermal regimes as supporting a
deep subsurface biosphere. For example, it has been suggested
that hydrogen produced from basalt-ground-water interactions may
serve as an energy source that supports the existence of
microorganisms in the deep subsurface of the Earth (Steven and
McKinley, 1995).
However, Anderson et al., (1998) have
demonstrated experimentally that hydrogen is not produced from
basalt at an environmentally relevant alkaline pH. Furthermore,
geochemical considerations suggest that previously reported
rates of hydrogen production couldn’t be sustained over
geologically significant time frames. Nevertheless, results from
the Anderson et al., (1998) study do not rule out the
possibility that reduced gases emanating from deeper in the
Earth could fuel deep subsurface microbial ecosystems (Gold,
1992).
Finally, the hypothesis that a reducing lithosphere on
the early Earth would have resulted in an ammonia-rich
atmosphere was tested experimentally by using a mineral catalyst
to reduce N2, NO2 - and NO3 - to ammonia (NH3) under typical crustal
and oceanic hydrothermal conditions (Brandes et al., 1998).
Results of this study showed that oceanic hydrothermally derived
ammonia could have provided the reservoir needed to facilitate
the synthesis of these compounds on the early Earth.
All of these studies indicate that a direct evaluation of the
subsurface biosphere ecosystem is needed to assess the
plausibility that organic syntheses capable of supporting life
can occur in this environment. A planned program to sample
water, porewater and sediment samples for the detection of
organic components (i.e. amino acids, peptides etc.) is
necessary to ascertain the mechanism of formation (abiotic or
biotic) and further determine whether the organic components
detected are capable of supporting or synthesizing a subsurface
biosphere.
These samples can be collected and examined on board
using conventional organic geochemical approaches (i.e. HPLC,
PY-GCMS, etc.). In addition, a planned re-entry program will
allow us to measure for organics in situ downhole (e.g.
state-of–the-art fiber-optic fluorescence or micro-Raman
approaches).
The use of fluorescence for the detection of
organic compounds is an extremely versatile and sensitive
technique (detection at the sub-femtomole level).
The development of an ‘Organic Probe’ that we can attach to a
re-entry device to detect organic components ‘real-time’ in the
Lake Vostok aqueous and sedimentary environment is needed. These
measurements are critical to the assessment of contamination
that may be introduced during the sampling program.
Thus, the
instrument implementation and the results obtained will be
important to future investigations of life in extreme
environments on Earth and perhaps beyond (e.g. Europa).
Hypotheses about the Lake Vostok Ecosystem
Diane McKnight
INSTAAR, University of Colorado, 1560 30th St., Boulder, CO
80309-0450, U.S.A.,
p (303) 492-7573, f (303) 492-6388,
Diane.McKnight@Colorado.edu
Lake Vostok allows us an opportunity to extend our knowledge of
ecosystem processes to a new extreme environment; one in which
there has been sufficient time for microorganisms to adapt.
Our
approach should be to develop ecosystem hypotheses based upon
current knowledge. Our current knowledge of environments of this
type is based on the Dry Valley ecosystem characteristics.
Dry Valley ecosystem characteristics:
1. Autotrophs in lakes and streams are adapted to use low
energy, e.g. photosynthesis begins with sunrise.
2. Relict organic carbon sustains ecosystems at a slow rate over
long periods, e.g. soil system runs on old algal carbon.
3. All landscape components - lakes, streams, soils - have a
food web, e.g. “microbial loop” in lakes.
4. In the lakes, viable organisms persist through winter and
mixotrophs become abundant.
Hypotheses about the Lake Vostok ecosystem:
1. Autotrophic microorganisms exist and use chemical energy
sources at very low fluxes.
2. The Lake Vostok ecosystem will be primarily heterotrophic,
with organic compound deposited with snow on plateau as an
organic carbon source.
3. The Lake Vostok ecosystem will have a microbial look,
including mixotrophs and grazers.
* Even if DOC of glacier ice is 0.1 mg C/L, this DOC may be a
greater energy source than those available to support
autotrophic processes. One could hypothesize that humics in Lake
Vostok water would have a different signature than humics in
overlying glacier ice because of microbial processing.
Plan for discoveries, for unexpected observations
It should be noted that studies in the Dry Valleys began in the
1960s, and were not conducted with a focus on avoiding the
introduction of exotic microorganisms. Although there is not
evidence of introduced algal species becoming abundant, we have
not assessed introductions as an ecological factor.
For isolated
inland locations, introductions should be a concern because
equipment or food could transport species that do not survive
long range aeolian transport.
A Terrestrial Analog
Mark Lupisella
NASA Goddard Space Flight Center, Greenbelt Rd., Mailstop 584.3,
Building 23, Rm. W207, Greenbelt, MD 20771, U.S.A.
p (301) 286-2918, f (301) 286-2325,
Mark.Lupisella@gsfc.nasa.gov
A key challenge for a human mission to Mars will involve
assessing and minimizing adverse impacts to the indigenous
environment, where “adverse” means anything that could
compromise the integrity of scientific research-especially the
search for life.
Due to the extreme surface conditions of Mars,
signs of Martian life, if they exist at all, are likely to be
under the surface where there is thought to be a layer of
permafrost.
It is also possible that sub-glacial lakes exist
under the polar caps of Mars.
Humans on Mars will eventually
have to drill for many reasons, including the search for life,
so Lake Vostok should be considered as a terrestrial analog for
understanding how humans might conduct such drilling activities
on Mars-particularly regarding issues of contamination control.
Microbial Sample Characterization and Preservation
David Emerson
American Type Culture Collection, 10801 University Blvd.,
Manassas, VA 20110-2209, U.S.A.,
p (707) 365-2700, f (707) 365-2730,
demerson@gmu.edu
Characterization and preservation of samples of microbes that
are returned from Lake Vostok will be a vital aspect of any
attempt to study the life that lives in the Lake.
The American
Type Culture Collection (ATCC) houses the world’s most diverse
collection of microorganisms, and includes large collections of
prokaryotes, fungi, and free-living protists. Members of all
these groups are likely to be found in Lake Vostok waters.
ATCC
scientists are well versed in the methods of cryopreservation
and lyophilization of microbes, and microbe containing samples,
as well as in isolation and characterization of the microbes
themselves. Recently, the ATCC has acquired the ability to carry
out more extensive genomic analysis of isolates, including
sequencing of SSU rRNA genes, DNA fingerprinting, and
hybridization technologies.
In addition, the ATCC has a strong
bioinformatics group with experience in developing databases
concerning specific groups of microorganisms. The ATCC would be
a willing participant in efforts to preserve and characterize
samples returned from Lake Vostok.
Some of the issues regarding sample handling from the Lake would
involve returning unfrozen samples through the ice sheet for
culturing. It is known that one freeze/thaw cycle can
significantly diminish the number of viable organisms in a
sample and can be especially hard on the protists.
An
alternative would be to inject cryopreservatives into samples in
situ so freezing upon return would be less deleterious, although
some protists will not tolerate any freezing at all.
Once
samples are returned to the surface, it will be important to
have the logistical support in place to insure that they remain
close to ambient temperature (assuming the ambient temperatures
are near 0°C, and not from a ‘hot spot’) during any transport
and handling back to the laboratories where they will be
processed. In addition, assuming samples are returned unfrozen,
it would be wise to preserve a subset of samples with different cryopreservatives for archival maintenance.
In terms of cultivating microbes, and especially novel
prokaryotes from Lake Vostok samples,
the most interesting habitat from a physiological perspective
would be the putative gas clathrates
that exist in the Lake. While methane clathrates are known to
exist at cold seeps in the Gulf of
Mexico, and other deep-sea environments, relatively little
microbiological work has been done
with these, and environments suitable for life containing other
types of gas clathrates are even less
known.
These unusual chemical conditions are most likely to lead
to unusual metabolic/phylogenetic types of microbes. Understanding and reproducing
the conditions whereby it might be possible to culture these
organisms will be important, and will require collaboration
between chemists and microbiologists to establish the best
methods.
It would be best to have the protocols for these
methods worked out prior to sample return; for cultivation
studies it is best to use ‘fresh’ samples and there may be a
relatively narrow window of a few weeks to have the highest rate
of success for cultivation.
Finally, from a culture collection
perspective, it would be ideal to have thorough documentation
procedures in place for any biological samples collected from
Lake Vostok.
This would include a WWW accessible database that
would catalog where samples were taken, how they were preserved,
where they were distributed, and a summary of the results
obtained for each sample, including the ultimate deposition of
any isolated microbes from the samples with a major culture
collection.
Ready access to this information would insure the
widest participation of the whole scientific community in what
is likely to be a highly unique and exciting, though costly,
endeavor.
Motivation for Sampling Hydrates and Sediments
Peter T. Doran
University of Illinois at Chicago, Department of Earth and
Environmental Sciences, 845 W. Taylor St., Chicago, Illinois,
60607-7059, U.S.A.
p (312) 413-7275 f (312) 413-2279,
pdoran@dri.edu,
pdoran@uic.edu
The impetus to study a deep subglacial lake such as Lake Vostok
will undoubtedly be driven by the investigation of life’s
extremes on this planet.
Extremes for life in Lake Vostok will
include high pressure (for a freshwater environment), low
nutrient levels, absence of light, and all gases being in
hydrated form.
Lake Vostok is analogous to the bottom 500 m of a
4 km deep freshwater lake with a 3.5 km perennial ice cover. The
motivation for studying Lake Vostok is similar to the motivation
for studying other unique and extreme habitats such as Antarctic
Dry Valley lakes, hydrothermal vents, and the deep Earth.
Defining modern life’s extremes is critical to understanding the
origins and evolution of life on this planet and others. Having
said this, science at Lake Vostok should not be limited to the
search for life. If no life exists in Lake Vostok we will want
to know why, which will require a detailed biogeochemical
sampling of the lake. Furthermore, the water column and
sediments of Lake Vostok should offer new and exciting sources
of paleoenvironmental information (e.g. CO2 clathrate record,
extraterrestrial flux), even in the absence of a viable lake
community.
The sediment record could conceivably extend well
beyond ice core records. The first stage of any Lake Vostok
study should be exploration with in situ instruments, but in
situ monitoring will fall short of answering the key science
questions (particularly in the sediment record).
Samples will
need to be brought to the surface, which appears feasible with
some technology development. Access and retrieval technologies
should be tested in a smaller, logistically convenient subglacial lake or analogous environment prior to going to
Vostok.
Some Factors Influencing Circulation in Lake Vostok
Eddy Carmack
Institute of Ocean Sciences, Institute of Ocean Sciences, 9860
West Saanich Rd.
P.O. Box 6000, Sidney BC V8L4B2, Canada,
p (250) 363-6585, f (250) 363-6746,
CarmackE@pac.dfo-mpo.gc.ca
Density-driven flows are likely to dominate water motion within
Lake Vostok. Hence,
consideration must be given to
(1) the equation of state of
fresh water
(2) the effect of pressure on
freezing point
(3) potential material flux from the overlying
ice
(4) geothermal heating from below
In turn, these
factors may be modified by sloping boundaries, e.g. along the
ice-water interface (ceiling) and water-sediment (floor) of the
lake. Some simple constraints follow from basic thermodynamic
considerations.
The depression of the temperature of maximum
density (TMD) with pressure is given by TMD(S, p) = TMD(0, p) -
0.021p, where p is pressure in bars or 105 Pa (Chen and Millero,
1986). The depression of the freezing temperature (TFP) with
pressure is given by TFP(S, p) = TFP(S, 0) ñ 0.00759p (Fujino et
al., 1975).
Taking TMD(0, 0) ~ 4oC and TFP(0, 0) ~ 0oC we see
that the two lines cross at a critical pressure (pcrit) of about
305 bars, which corresponds to an overlying ice thickness of
about 3350 m. Above this critical pressure TMD > TFP and the
system is stable when (T/(Z > 0); that is, it behaves as a lake.
Below this pressure TMD < TFP and the system is stable when
(T/(Z < 0); that is, it behaves as an ocean. It appears that
pressures with Lake Vostok place it in the “ocean” category.
Other Antarctic lakes, for example the one at South Pole, may
fall into the “lake” category.
An interesting situation would
arise if pcrit were to lie internal to the lake, yielding
bimodal flow conditions. External sources of buoyancy to the
system include geothermal heating (perhaps ~ 50 mWm-2) and
particle fluxes (unknown, but, if existent, likely to be highly
localized).
Lateral gradients of buoyancy may also arise from
boundary conditions at the sloping ceiling (required to be at
the local TFP) and bottom (derived from either geothermal
effects or solute flux). It is noted that examples are found
elsewhere in nature where extreme pressures affect water
stratification and motion; for example in the oceans off
Antarctic ice shelves (Carmack and Foster, 1975) and in deep
lakes such as Baikal (Weiss et al., 1991).
Prior to in situ
measurements of circulation in Lake Vostok, possible scales of
motion should be explored with simple models.
Also, field
experiments could be carried out to see if flow can be detected
in similar but less extreme high pressure and low temperature
situations (e.g. beneath the Ward Hunt Ice shelf off Ellsmere
Island (Jefferies, 1992).
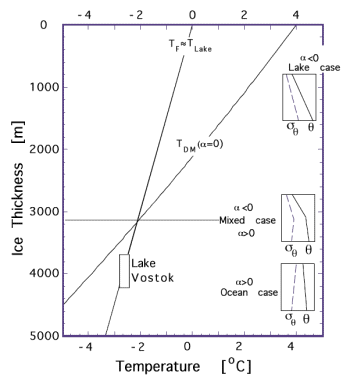
Figure Caption
Concerning water column stratification, three types of lakes
under ice can be expected in Antarctica,
depending on whether the ice thickness is larger or less than
the depth, where freezing temperature TF and
the temperature of maximum density TMD are identical (3170 m
ice).
Lake Vostok, where TF (lake
temperature = -2.7oC) is warmer than TMD ( -4oC), thermal expansivity
a
is positive and subsequently
density sT decreases with depth, as typical under convective
instability.