
by Wayne Schmidt
from
ThisAndThat Website
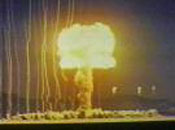
While the image above is the
stereotypical picture of a nuclear explosion, in reality by the time
the classic mushroom cloud has formed all the interesting detail is
long over.
The following images, borrowed from several sources, show
the eerie and complex patterns atomic detonations create immediately
after they are triggered. Most of the following images were taken
using Rapatronic cameras, ultra-high speed, single-frame cameras
developed in the 1940s by Dr.
Harold Edgerton.
The duration of the
exposure is typically 10 nanoseconds (0.00000001 of a second. That's
so short that light, which can travel a distance equal to 7 times
around the earth in 1 second, would only cover 98 feet.)
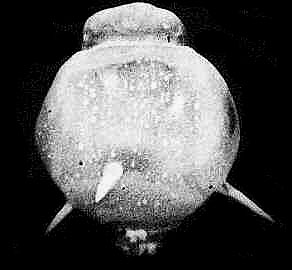
This image captures two common elements:
At this stage of the detonation the surface of the fireball has a
temperature of 20,000 degrees, three times hotter than the sun's
surface. At such temperatures the amount of thermal radiation
(light) given off is so enormous anything it touches is vaporized
ahead of the expanding fireball. The three spikes in above image
result from the guide wires supporting the tower on which the bomb
was located absorbing enough heat to turn into light emitting
plasma. Because thermal radiation travels faster than the fireball,
the spikes extend out ahead of it.
Experiments with different support wires showed that if they were
painted black to better absorb radiation they were longer. If
painted with a reflective silver paint they don't appear at all.
One might expect an explosive fireball to expand in a perfect
sphere. Actually, variations in the density of the bomb's
surrounding case create the mottlings and and complex shapes in many
of these images.
The support tower in the image above provides a convenient size
scale. Most of the above images capture the fireball when it is 100
feet in diameter (33 meters about), typically 0.001 seconds after the control operator
pressed the "fire" button.
The image above was taken 20 milliseconds later than the others and
shows the fireball expanded to 300 feet in diameter, the size of a
football field. Instead of a sphere, the fireball has become large
enough so that its bottom is in contact with the earth. The even,
light grey ring near the bottom of the smooth fireball (more
properly called a firedome in this case) is the convolution of the
shock wave from the fireball and the reflection of that shock wave
from the surface of the earth.
This doubly-enhanced shock wave is
the area of maximum destructive force, as shown by the expanding
ring of rubble below it. For any given bomb size, there is an
optimum altitude at which to detonate it to make use of this
reflected shock effect to maximize damage.
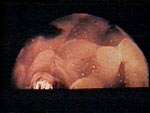
The previous images were of smaller nuclear devices measured in tens
to hundreds of kilotons. The one immediately above was taken of the
Ivy MIKE device with a rating of 10.7 megatons.
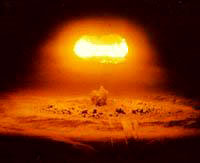
The typical mushroom cloud forms when the fireball occurs near to
the earth, then rises pulling a smoke cloud in its wake. In high
aerial blasts, like this final image, the detonation altitude is
high enough that there is a break between the "cap" of the mushroom
and the earth.
Additional
Trivia
-
The surface of the fireball
initially expands outward at a rate of tens of thousands of
meters per second.
-
During the early years of nuclear
research, the main effort was in creating larger and more
powerful bombs, many of which were rated at several tens of
megatons. However, military experts quickly discovered that
since the energy from a detonation expands in a sphere, much of
the energy is wasted at high altitudes. The optimum size, in
terms of the most destructive power from the smallest amount of
fissionable material, comes from bombs in the 100-kilotons
class.
-
The average US bomb measures 250
kilotons.
-
The average Russian bomb measures
400 kilotons
-
In comparison, the bomb dropped on
Hiroshima was 15 kilotons and the one dropped on Nagasaki was 21
kilotons.
-
A nuclear detonation occurs when
enough fissionable material (called the critical mass) is
brought together quickly enough to cause a runaway chain
reaction. For uranium 235 this is around 22 pounds.
-
Nuclear fuel is unbelievably energy
dense. The volume of plutonium used in the Nagasaki bomb was the
size of a softball. Even more amazing is that the 21 kiloton
yield reflects a very inefficient use (a few percent) of the
total energy potential of the plutonium.
-
Nuclear explosions are possible from
smaller amounts of fissionable material by using the extremely
high pressure of a surrounding chemical explosion to compress
fissionable material to such a high density that a runaway chain
reaction can be sustained.
-
Nuclear detonations all experience a
characteristic "double flash." Early in the detonation the
explosion consists of two concentric spheres. The outer one is
created by a sudden flood of radiation being absorbed by the
surrounding air and heating it to incandescence. The inner one
is the fireball of the vaporized solid elements of the bomb
itself. The ionized structure of the outer shell makes it opaque
to light from the inner shell so all that can be seen is the
light the outer shell gives off. A millisecond or so after the
detonation, the inner shell expands far enough that it blows
through the outer shell and the glowing surface of the now
exposed inner fireball can be seen, hence the second flash.
-
The largest nuclear weapon ever
exploded was Russia's "super-bomb," rated at 60 megatons.
-
The problem with getting a nuclear
bomb to explode is bringing the fissionable materials together
fast enough. Done too slowly they melt and vaporize before the
runaway chain reaction can take place. The required rapid
combination is achieved by surrounding the core segments with
chemical explosives. While these explosives create the speed of
combination required, they also create another problem: uneven
detonation.
To get a sphere of explosives to
detonate all at once many detonators have to be used. These have
to be timed to all go off at exactly the same time. Doing so
required numerous problems such as variations in detonator
reaction time, delays caused by detonator cable lengths, uneven
local explosion forces caused by minor variations in the
explosive and a host of other issues to be solved. Think of
trying to wrap jello with rubber bands and you get a feel for
the problem.
In theory it's possible, but doing
it in the real world is a challenge.
Making an atomic bomb is not the
simple task it's made out to be in television thrillers.
|