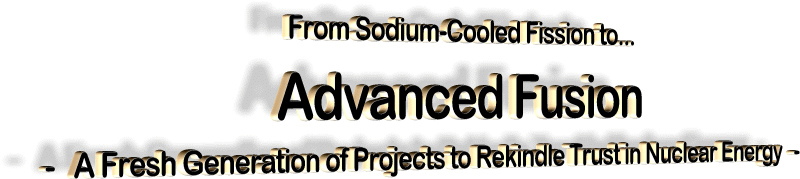
by Leigh Phillips
February
27, 2019
from
TechnologyReview Website
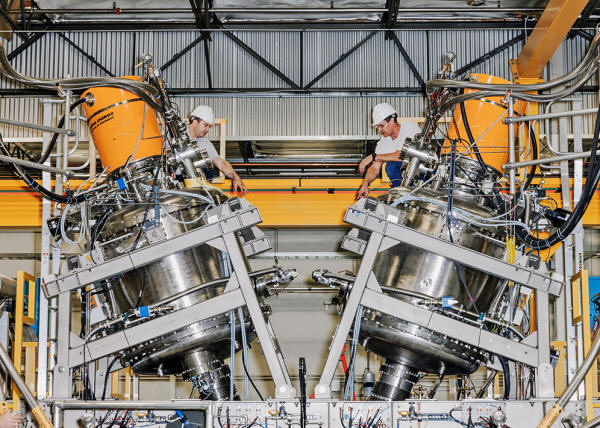
A photograph taken in 2016
shows the central confinement vessel
of a prototype fusion reactor
built by Tri Alpha Energy
(now TAE Technologies).
Julian Berman
The new, safer
nuclear reactors
that might help
'stop' climate
change...
BP might not be the first source you go to for environmental news,
but its annual energy review is highly regarded by climate watchers.
And its 2018 message was
stark:
despite the angst over global warming, coal was responsible
for 38% of the world's power in 2017 - precisely the same level as
when the first global climate treaty was signed 20 years ago.
Worse still,
greenhouse-gas emissions rose by 2.7% last year, the largest
increase in seven years.
Such stagnation has led many policymakers and environmental groups
to conclude that we need more nuclear energy.
Even United Nations
researchers, not enthusiastic in the past, now say every plan to
keep the planet's temperature rise under 1.5°C will rely on a
substantial jump in
nuclear energy.
But we're headed in the other direction.
And even if nuclear had
broad public support (which it doesn't), it's expensive:
several
nuclear plants in the US closed recently because they can't compete
with cheap shale gas.
"If the current
situation continues, more nuclear power plants will likely close
and be replaced primarily by natural gas, causing emissions to
rise," argued the
Union of Concerned Scientists - historically
nuclear skeptics - in 2018.
If all those plants shut
down, estimates suggest, carbon emissions would increase by 6%.
At this point, the critical debate is not whether to support
existing systems, says Edwin Lyman, acting director of the UCS's
nuclear safety project.
"A more practical
question is whether it is realistic that new nuclear plants can
be deployed over the next several decades at the pace needed."
As of early 2018 there
were 75 separate advanced fission projects trying to answer that
question in North America alone, according to the think tank
Third
Way.
These projects employ the
same type of reaction used in the conventional nuclear reactors that
have been used for decades - fission, or splitting atoms.
One of the leading technologies is the small modular reactor, or
SMR:
a slimmed-down
version of conventional fission systems that promises to be
cheaper and safer.
NuScale Power, based in
Portland, Oregon, has a 60-megawatt design that's close to being
deployed (a typical high-cost conventional fission plant might
produce around 1,000 MW of power.)
NuScale has a deal to install 12 small reactors to supply energy to
a coalition of 46 utilities across the western US, but the project
can go ahead only if the group's members agree to finance it by the
end of this year.
History suggests that
won't be easy.
In 2011,
Generation mPower, another SMR developer,
had a deal to construct up to six reactors similar to NuScale's. It
had the backing of corporate owners
Babcock & Wilcox, one of the
world's largest energy builders, but the pact was shelved after less
than three years because no new customers had emerged.
No orders meant prices
wouldn't come down, which made the deal unsustainable.
While NuScale's approach takes traditional light-water-cooled
nuclear reactors and shrinks them, so-called generation IV systems
use alternative coolants.
China is building a large
scale
sodium-cooled reactor in Fujian province that's expected to
begin operation by 2023, and Washington-based
TerraPower has been
developing a sodium-cooled system that can be powered with spent
fuel, depleted uranium, or uranium straight out of the ground.
TerraPower -
Bill Gates
is an investor - forged an agreement with Beijing to construct a
demonstration plant by 2022, but the
Trump administration's
restrictions on Chinese trade make its future questionable.
Another generation IV variant, the
molten-salt reactor, is safer
than earlier designs because it can cool itself even if the system
loses power completely.
Canadian company
Terrestrial Energy plans to
build a 190 MW plant in Ontario, with its first reactors producing
power before 2030 at a cost it says can compete with natural gas.
One generation IV reactor could go into operation soon.
Helium-cooled, very-high-temperature reactors can run at up to
1,000°C, and the state-owned China National Nuclear Corporation has
a 210 MW prototype in the eastern Shandong province set to be
connected to the grid this year.
Three reasons for renewed hope for nuclear power
|
Small
modular reactors |
Advanced fission |
Fusion |
|
SMRs are a
slimmed-down version of conventional fission
reactors. Although they produce far less
power, their smaller size and use of
off-the-shelf components help reduce costs. |
These reactors
are designed to be safer than traditional
water-cooled reactors, using coolants such
as liquid sodium or molten salts instead.
Most advanced is the “pebble bed” reactor,
cooled by a gas such as helium; China is
ready to connect the first such reactor to
the grid this year. |
Technical
progress is still slow after decades of
investment, but fusion companies are focused
on how to contain the plasma required to
replicate the thermonuclear conditions of
the sun. Techniques include magnetic
confinement, which traps plasma continuously
at low pressure; inertial confinement, using
lasers and pulsing plasma for nanoseconds at
a time; and magnetized target fusion, which
combines the two with pulses of plasma
controlled by magnets. |
Companies |
NuScale Power
|
China National
Nuclear Corporation, TerraPower, Terrestrial
Energy |
ITER, TAE
Technologies, General Fusion, Commonwealth
Fusion Systems |
Power
output |
50-200
megawatts |
190-600
megawatts |
100-500
megawatts |
Expected life span |
60 years |
40-60 years |
35 years |
Cost |
$100 million
prototype
$2 billion to develop |
Pebble beds:
$400 million to $1.2 billion
Sodium-cooled
and molten salt: $1 billion prototype |
ITER:
currently $22 billion
Cost of a commercial version is unknown |
Available |
2026 |
Pebble bed in
2019; sodium-cooled 2025; molten salt 2030 |
No earlier
than 2035 |
|
For many, though, the great energy hope remains nuclear fusion...
Fusion reactors mimic the
nuclear process inside the sun, smashing lighter atoms together to
turn them into heavier ones and releasing vast amounts of energy
along the way.
In the sun, that process is powered by gravity.
On
Earth, engineers aim to replicate fusion conditions with
unfathomably high temperatures - on the order of 150 million°C -
but they have found it hard to confine the plasma required to fuse
atoms.
One solution is being built by
ITER, previously known as the
International Thermonuclear Experimental Reactor, under construction
since 2010 in Cadarache, France.
Its magnetic confinement system has
global support, but costs have exploded to $22 billion amid delays
and political wrangling.
The first experiments, originally scheduled
for 2018, have been pushed back to 2025.
Vancouver's
General Fusion uses a combination of physical pressure
and magnetic fields to create plasma pulses that last millionths of
a second.
This is a less complicated approach than ITER's, making it
far cheaper - but technical challenges remain, including making
titanium components that can handle the workload.
Still, General
Fusion expects its reactors to be deployable in 10 to 15 years.
California-based
TAE Technologies, meanwhile, has spent 20 years
developing a fusion reactor that converts energy directly into
electricity. The company, which has received $500 million from
investors, predicted in January that it would be commercial within
five years.
Many voters simply
don't believe companies' promises
that new
technologies
can avoid old mistakes...
So will any of these technologies succeed...?
Advanced fission reduces
nuclear waste - even using it as fuel - and drastically shrinks the
chance of tragedies
like Fukushima or Chernobyl. Yet no such
reactors have been licensed or deployed outside China or Russia.
Many voters simply don't believe companies when they promise that
new technologies can avoid old mistakes.
It's not just politics, though:
cost is also a factor.
Advanced
fission promises to reduce the ridiculously expensive up-front costs
of nuclear energy by creating reactors that can be factory built,
rather than custom made.
This would cause prices to plummet, just as
they have for wind and solar.
But private companies have rarely
proved successful at bringing these projects to completion:
the
biggest advances have come from highly centralized, state-driven
schemes that can absorb risk more easily.
General Fusion CEO Chris Mowry argues that fission simply faces too
many barriers to be successful.
He has experience:
he was a founder
of mPower, the SMR company that was mothballed in 2014. Fusion
reactors might be harder to build, he suggests, but they are more
socially acceptable.
This is why there's been a rush of venture
capital into fusion, he says - investors are confident there will be
a sea of eager buyers waiting for whoever can make it work first.
But does fusion really have that much more room to maneuver?
It's
true that the low-level, short-lived radioactive tritium waste it
produces represents no serious danger, and the technology means that
meltdowns are impossible.
But costs are still high
and time lines are still long:
ITER's fusion reactor is massively more expensive
than originally planned and won't be workable for at least 15 years.
Meanwhile, Green 'politicians' in Europe already want ITER shut down,
and many anti-nuclear campaigners don't distinguish between fission
and fusion:
-
nuclear fusion
is a reaction in which two or more atomic nuclei are
combined to form one or more different atomic nuclei and
subatomic particles (neutrons or protons). The difference in
mass between the reactants and products is manifested as
either the release or absorption of energy. This difference
in mass arises due to the difference in atomic "binding
energy" between the atomic nuclei before and after the
reaction. Fusion is the process that powers active or "main
sequence" stars, or other high magnitude stars.
Source
-
nuclear
fission is a nuclear reaction or a radioactive decay
process in which the nucleus of an atom splits into smaller,
lighter nuclei (like in Fukushima or Chernobyl). The
fission process often produces free neutrons and gamma
photons, and releases a very large amount of energy even by
the energetic standards of radioactive decay.
Source
Experts might be lining up behind nuclear, but convincing skeptical
voters is something else...
|