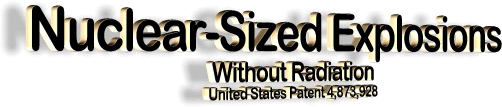
from
UnitedStatesPatentAndTrademarkOffice
Website
Abstract
A method for producing a high yield explosion without radioactive
fallout comprising filling an expendable structure with an explosive
mixture of a combustible gas (e.g. methane) and an oxidizer gas
(e.g. oxygen) and then detonating said mixture.
- United States Patent:
4,873,928
- October 17, 1989
-
Inventors: Lowther; Frank E. (Plano, TX) -
Assignee: APTI, Inc. (Los Angeles, CA)
-
Appl. No.: 07/062,020 -
Filed: June 15, 1987
-
Current U.S. Class: 102/323 ; 102/324; 149/109.2; 434/218
-
Current International Class: F42D 1/10 (20060101); F42D 1/00
(20060101); F42D 3/00 (20060101); F42B 3/087 (20060101); F42B 3/00
(20060101); F42B 12/02 (20060101); F42B 12/52 (20060101); F42B
003/00 ()
-
Field of Search: 149/109.6,109.1,109.2 102/323,324 434/218
-
References Cited [Referenced By]
-
Other References:
Austin et al., Explosive Hazard of Aluminum-Liquid Oxygen Mixtures,
Jour. Chem. Ed., vol. 36, #2, Feb. 1959, pp. 54-57..
-
Primary Examiner: Nelson; Peter A. -
Attorney, Agent or Firm: Faulconer; Drude
Claims
What is claimed is:
-
A method for producing a violent
and destructive explosion having high shock energies
comprising:
-
partially filling an
expendable structure with an initial mixture of
combustible gas and an oxidizer gas in concentrations
rich in said combustible gas so that said initial
mixture is incapable of burning or exploding;
-
mixing additional oxidizer
gas into said initial mixture after said initial mixture
is in place and contained within said structure to
thereby create an explosive mixture within said
structure; and
-
detonating said explosive
mixture to produce said explosion and thereby destroying
said expendable structure.
-
The method of claim 1 wherein
said combustible gas is comprised of methane.
-
The method of claim 1 wherein
said combustible gas is comprised of a mixture of methane
and hydrogen.
-
The method of claim 2 wherein
said oxidizer gas is comprised of oxygen.
-
The method of claim 4 wherein
said additional oxidizer gas is mixed with said initial
mixture by flowing oxygen gas into said structure after said
initial mixture is in place within said structure.
-
The method of claim 4 wherein
said step of mixing additional oxidizer gas into said
initial mixture comprises:
-
positioning a container of
liquid oxygen within said initial mixture within said
structure;
-
positioning an explosive
means within said container; and
-
detonating said explosive
means to vaporize and disperse said liquid oxygen into
said initial mixture.
-
The method of claim 1 wherein
said explosive mixture is detonated by exploding
conventional explosives positioned within said structure.
-
The method of claim 1 including:
-
The method of claim 8 wherein
said dust-like particles are comprised of aluminum.
-
The method of claim 1 wherein
said explosive mixture is detonated by exploding bridge
wires positioned within said structure.
-
The method of claim 1 including:
-
positioning a mesh of
metallic, wire-like filaments throughout said structure;
and
-
igniting said mesh to
detonate said explosive mixture.
-
The method of claim 1 wherein
said mesh is comprised of zirconium.
-
The method of claim 1 wherein
said structure is comprised of an inflatable envelope.
-
The method of claim 13 wherein
said envelope is spherical in configuration when fully
inflated.
-
The method of claim 1 wherein the
energy yield of said explosive is equivalent to one kiloton
or greater of T.N.T.
DESCRIPTION
1. Technical Field
The present invention relates to a method for producing
explosions from an explosive gas mixture which has a shock yield
comparable to a nuclear explosion but one which produces no
radioactive fallout.
2. Background Art
Between 1945 and 1960, the United States exploded nuclear
devices which had a total yield equivalent to approximately 200
megatons (MT) of trinitrotoluene (T.N.T.). Tests conducted by
other countries during this time brought the total yield of
nuclear explosions to approximately 400 MT. It was quickly
recognized that such testing could not continue since each
nuclear explosion produced radioactivity that seriously
threatened the environment. To protect the environment from such
radioactive fallout, most of the world's nuclear powers signed
the Nuclear Test Ban Treaty in 1963 which prohibited nuclear
explosions in the atmosphere, underwater, and in space. Since
that time, it is believed that substantially all nuclear
explosions have been carried out underground.
The necessary prohibition against above-ground testing of
nuclear explosives, however, has created situations where
alternates to such explosions are needed. For example, all of
the sophisticated communication systems, defense systems, weapon
systems, etc. that have been designed and built for the military
since 1963 have never been tested in their ultimate operating
environments, i.e. under nuclear blast conditions. Obviously,
any such testing has to be simulated through theoretical studies
and/or under laboratory conditions.
Radiation dosages and
electromagnetic pulses which simulate those from nuclear
explosions have been produced in shielded laboratories but the
extreme shock energies which can be expected from nuclear
explosions, e.g. 1 kiloton (KT) or larger, have not been
satisfactorily duplicated. Without realistic testing, the
question will always remain as to whether or not a particular
system or component will survive a nuclear explosion. To provide
such realistic testing with conventional explosions is
impractical as will become obvious from the following
discussion.
The conventional explosive, T.N.T., is the recognized standard
of measurement and comparison for both nuclear and non-nuclear
explosions. That is, the exploding of a kiloton (KT) or
2,000,000 pounds of T.N.T. releases 4.1.times.10.sup.9 Btus. It
follows that the exploding of any material that release
4.1.times.10.sup.9 Btus is referred to as a 1 KT explosion. To
amass 1 KT of T.N.T. at a single test site, in itself, is an
ambitious and dangerous undertaking. For example, it is
estimated that it would take a 20-boxcar train to transport this
amount of T.N.T. and the risks involved with such a shipment
from a manufacturing or storage facility to the test site are
self evident.
Further, when detonated, one volume of T.N.T. suddenly converts
to 1000 volumes of gas. The speed and uniformity of "burn" and
therefore the violence of T.N.T. depends upon the uniformity of
the ignition method. Normal sized T.N.T. charges explode in
microseconds but massive T.N.T. charges may take much longer due
to the practical problems involved in uniform ignition. The
transportation and the uniform detonation problems of large
masses of T.N.T. makes its use as a source for simulated,
nuclear-sized explosions both unattractive and impractical.
Accordingly, to produce a practical, non-nuclear explosion
having nuclear-sized shock yields, the following criteria would
seem imperative. First, there must be a reliable source of an
explosive which is readily available in adequate quantities to
support a continuing test program. Next, the explosive must be
capable of being safely and reliably transported from its source
to a remote test site. Further, a relatively inexpensive,
expendable, test structure must be provided in which the
explosive can be loosely contained until detonated.
Lastly, the
explosive must be capable of relatively instant and uniform
detonation so that the violence of the blast adequately
simulates that of a nuclear explosion.
DISCLOSURE OF THE
INVENTION
The present invention provides a method for producing an explosion
which yields shock energies equivalent to nuclear explosions but one
which generates no radioactive fallout.
Basically, the present
invention utilizes a commonly available combustible gas, e.g.
natural gas, methane, etc., mixed with a commonly-available oxidizer
gas, e.g. oxygen, air, etc., as the explosive mixture for a large
scale explosion. Methane, e.g. natural gas, is readily available in
large quantities which can quickly and easily be transported to a
remote test site by a common pipeline or the like.
More specifically, the present invention provides a method for
producing an explosion by filling a large, expendable structure,
e.g. an inflatable envelope, at a test site with an explosive
mixture of methane and oxygen and then detonating said mixture. To
provide a relatively safe operating environment during the filling
operation, the envelope is first partially filled with an initial
filling mixture comprised of methane and oxygen wherein the
concentration of methane is too rich for the mixture to either burn
or explode.
After the initial filling mixture is in
place, the mixture is "armed" or "topped-off" with additional
oxidizer gas, e.g. oxygen, either in gas or liquid form, to change
the relative concentration of methane and oxygen to one which will
readily explode when detonated.
A plurality of detonation means can be used to effect a relatively
uniform detonation front across the mixture within the envelope.
Such means include conventional explosives, exploding bridge wires,
dipole antennae activated by radio frequencies, metallic or organic
dust particles, and/or metallic filaments dispersed throughout the
explosive mixture.
BRIEF
DESCRIPTION OF THE DRAWINGS
The actual construction, operation, and the apparent advantage of
the invention will be better understood by referring to the drawings
in which like numerals identify like parts and in which:
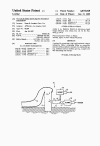
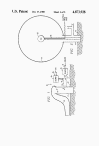
Fig 1-2 |
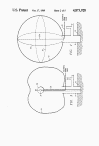
Fig 3-4 |
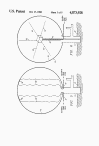
Fig 5-6 |
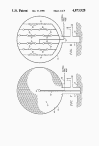
Fig 7-8 |
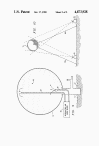
Fig 9-10 |
FIG. 1 is a schematical
section of a test site having apparatus for inflating an envelope
with a combustible gas-oxidizer gas mixture
FIGS. 2-9 are schematical
sections of a variety of different means for detonating an explosive
gas mixture within the envelope of FIG. 1
FIG. 10 is an illustration of
the envelope of FIG. 1 at a position above the surface
BEST MODE FOR
CARRYING OUT THE INVENTION
Referring more particularly to the drawings, FIG. 1 (far
below) discloses a test
stand 10 comprised of base conduit 11 which is anchored into the
ground 12 by any suitable means. A gas-tight, expendable, test
structure, e.g. flexible envelope 13, has a filling inlet 14 which
is secured in a fluid-tight relationship over the upper end of
conduit 11.
Conduit 11 has a manifold 15 connected thereto which, in
turn, has two inlets 16, 17. Inlet 16 is connected to a combustible
gas blower 18 while inlet 17 is connected to an oxidizer gas blower
17.
Preferably, the combustible gas is one that is naturally-available
in large quantities and is one that can easily and safely be
transported to the test site. For example, large quantities of
natural gas, i.e. methane, are readily available in several
geographical areas. Especially attractive are the huge quantities of
methane in the North Slope Area of Alaska since there are remote
areas nearby which are suitable as a test site. The methane can be
produced and safely transported to the test site by a common
pipeline.
However, the test site does not have to
be in close proximity to the methane production area since the
methane can be gathered and safely pipelined for long distances as
is now commonly done in supplying energy to areas remote from the
production area. In the following descriptions, methane will be used
in interchangeable with combustible gas and oxygen with oxidizer gas
but, it should be understood, that obvious equivalents of these
gases are intended to be covered hereunder.
A methane-oxygen mixture will neither burn nor explode if the
methane concentration (CH.sub.4) is less than 5.4% or greater than
59.2% by volume. Accordingly, blower 18 feeds methane from pipeline
29 and blower 19 feeds oxygen from line 21 into manifold 15 at rates
whereby the resulting mixture in manifold 15 is comprised of
approximately 59.2% methane and 40.8% oxygen by volume.
This initial filling mixture, which will
neither burn nor explode, then flows into envelope 13 to fill the
envelope to approximately 85% of its total capacity. By using a
non-explosive gas mixture to initially fill the envelope to near its
capacity, the test system remains "unarmed" and the risk of a
disastrous accident during filling is minimized. Also, the
partially-filled envelope can be left in this condition for extended
periods with little risk.
Just before a test explosion is to be carried out, the
methane-oxygen mixture in envelope 13 is armed or "topped-off" by
flowing only oxygen through manifold 15 until envelope 13 is filled
to capacity. At this time, the mixture in envelope 13 will be
comprised of approximately 50% methane and 50% oxygen, which is a
highly explosive mixture. A detonation means within envelope 13 (not
shown in FIG. 1) is actuated and a violent explosion results. The
means for detonating the gas mixture may any one of the several
means which are described in detail below.
To more fully understand the present invention, a specific example
for producing a non-nuclear explosion having a shock yield
equivalent to 1 KT of T.N.T. will now be set forth. A base conduit
11 having a diameter of 9.5 feet is securely anchored in ground 12.
A 110-horsepower (h.p.) blower 18 supplies methane through inlet 16
(7.3 feet diameter) into manifold 15 at a rate of approximately 2100
cubic feet per second (CF/sec) while a 75-h.p. blower 19 supplies
oxygen through inlet 17 (6 feet diameter) into manifold 15 at a rate
of approximately 1420 CF/sec.
To assure proper gas mixing and agitation, the mixture (59.2%
methane-40.8% oxygen by volume or CH.sub.4+ 0.689 0.sub.2) flows
into envelope 13 as an initial filling mixture at a linear velocity
of 50 feet/sec. Inflatable envelope 13 is comprised of DuPont 6/6
reinforced Nylon having a thickness of 2.42 mils. The reinforced
Nylon will not rupture if punctured, as a rubber ballon would, but
instead will only allow the gas to leak slowly through the puncture
itself.
When completely inflated, envelope 13 is
spherical with a diameter of 305 feet and a total capacity of
14.9.times.10.sup.6 CF. When empty, envelope 13 will weigh
approximately 1.29 tons. It will take approximately one hour at the
above rates to fill envelope to approximately 85% of its total
capacity with the non-explosive mixture. At this point, envelope 13
contains 12.6 million cubic feet (MMCF) of gas mixture of which
approximately 7.5 MMCF is methane and 5.1 MMCF is oxygen.
Preferably, a short time before the test is to be conducted, the
mixture is envelope 13 is "armed" or topped-off with additional
oxidizer gas, oxygen, to provide a highly explosive mixture of
approximately 50% methane-50% oxygen. To accomplish this,
approximately an additional 2.32 MMCF of oxygen is supplied through
manifold 15 by blower 19 at approximately 1420 CF/sec. In
approximately 27 minutes, envelope 13 will be fully inflated with
the explosive methane-oxygen mixture and will have an internal
pressure of approximately 0.1 psi above the ambient pressure. The
mixture is then detonated by any of the means described below.
It is estimated that when a 50% methane-50% oxygen mixture explodes,
1 MMCF of mixture yields energy equivalent to 0.0671 KT of T.N.T.
Accordingly, the 14.9.times.10.sup.6 CF volume within envelope 13
will yield energy equivalent to 1 KT of T.N.T.
The speed and uniformity of "burn" and therefore the violence of any
explosion depends upon the uniformity of the detonation method used
in initiating the explosion. If all of the available chemical energy
is to be released in an explosion, the combustion front must
propagate across the entire combustible gas volume without being
extinguished. It is the chemical heat release in the combustion
front that generates and supports a faster shock front. If the
combustion front is stopped or interrupted for any reason, the
explosion will stop or will be interrupted.
Turning now to FIGS. 2-8, various means for detonating the explosive
mixture in envelope 13 are disclosed.
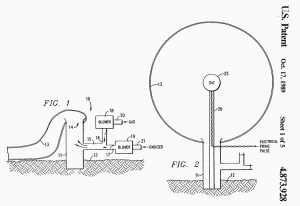
Fig 1-2
In FIG. 2, a charge 25 of a
conventional explosive such as T.N.T. is positioned at the
approximate center of envelope 13 on a support 26 and is sized to
provide rapid detonation of the volume of gas in envelope 13. Charge
25 may be prepositioned within the envelope before inflation begins.
Charge 25 is detonated in a conventional manner by an electrical
firing pulse through line 26.
FIG. 3 illustrates a modification of the present invention wherein
the test system is both armed (i.e. topped-off with oxygen) and
detonated substantially simultaneous. Envelope 13 is partially
inflated with a non-explosive, methane-oxygen mixture in the same
manner as described above. A charge 28 of conventional explosive,
e.g. T.N.T., is positioned within container 29 which is positioned
at the center of envelope 13 on support 30 and which is filled with
additional oxidizer gas.
The additional oxidizer gas is
preferably in concentrated form, e.g. compressed oxygen gas on
liquid oxygen (LOX) 31. If LOX is used, charge 28 is sized so that
when detonated by an electric pulse through line 32, the LOX will be
vaporized to provide and disperse the "arming" oxygen throughout the
mixture in envelope 13.
For the specific example described above, a
charge 28 of 9500 pounds of T.N.T. in a spherical shape having a
diameter of 5.7 feet is positioned within container 29 having a
diameter of 17.9 feet which, in turn, is filled with 103.5 tons of
LOX.
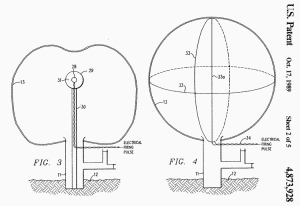
Fig 3-4
These amounts are calculated knowing the
heat of vaporization of LOX to be 92 Btus/pound and that the
exploding T.N.T. will provide 2000 Btus/pound.
FIG. 4 discloses another detonation means for initiating the
explosion of the methane-oxygen mixture in envelope 13. Lengths of
detonating cord 33, e.g. Ensign-Bickford "Primacord" by DuPont, is
secured to the inner surface of envelope 13 along various
circumferences (only two shown). The cords 33 are secured in
position before inflation and additional lengths 33a (only one
shown) can be suspended from the inside of the top of envelope so
that they will hand downward through the mixture when envelope 13 is
inflated.
Detonating cord is a flexible cord that
contains a center core of a conventional explosive, e.g.
pentaerythritol tetranitrate (PETN), which detonates at a velocity
of 22,000 feet per second and may contain up to 400 grains of
explosive per foot, enough to trigger an explosion. Cords 33, 33a
are detonated by a conventional detonator, e.g. blasting caps, which
is actuated by an electrical pulse through line 34.
FIG. 5 discloses still another means for detonating the mixture in
envelope 13. A plurality of bridge wires 35 (only two shown) are
looped within envelope 13 and attached at the inside of the upper
surface thereof before inflation so that they will extend across the
envelope when it is inflated.
Investigations into the use of
exploding bridge wires have revealed some very unique features
thereof such as the capability of:
(1) injecting energies in the 10
kilocalories and greater per mole range in submicrosecond
time intervals
(2) producing high energy which,
in turn, imparts high velocity to the physical mass of the
reactants in contact with the wire.
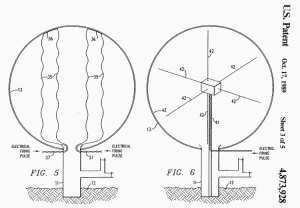
Fig 5-6
Accordingly, an exploding wire provides
a way of concentrating large amounts of energy in a small space.
Typically, a bank of capacitors (not shown) are charged to a high
voltage level. The capacitors are then discharged through lines 37
into wires 35. The current through the wires is many magnitudes
greater than that necessary to fuse the wire.
The extreme resistive heating in the
wire causes a mean instantaneous vaporization of the wire which
creates a shock wave in the atmosphere surrounding the wire to
thereby initiate an explosion of the mixture in envelope 13.
FIG. 6 discloses a still further means for detonating the explosive
mixture in envelope 13. A one-shot, radio frequency transmitter 40
is positioned at the center of envelope 13 on support 41. A
plurality of center-fed, dipole antennae 42 extend outward to the
inner surface of envelope 13 which may be coated with a thin
metallic layer 43, e.g. aluminum.
A single extremely high energy
radio-frequency pulse is delivered to transmitter 40 through line 43
which, in turn, transmits the energy through dipole antennae 42.
Since the energy is several orders of magnitude higher than antennae
42 are designed to handle, antennae 42 and metallic layer 43 will
vaporize, basically in the same manner as an exploding bridge wire
as described above.
FIG. 7 discloses another means for detonating the explosive mixture
in envelope 13. A center electrode 44 is positioned at the center of
envelope 13 on support 45 and the inner surface of envelope 13 is
coated with a thin metallic layer 46, e.g. zirconium, to act as a
second electrode. A mesh of thin wire-like, filaments of metal, e.g.
zirconium, is positioned within and throughout envelope 13 to create
what is, in effect, a giant "flash bulb".
A high energy, electrical
pulse is supplied through lead 48 to ignite the metallic mesh 47
throughout envelope 13 to thereby initiate the explosion.
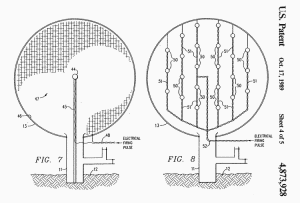
Fig 7-8
FIG. 8 discloses still another means for detonating the mixture in
envelope 13. A plurality of electric blasting caps 50 are spaced on
stringers 51 which are suspended within envelope 13 and are all
connected to a firing lead 52. Caps 50 are fast-functioning, high
strength instantaneous caps that detonate in less than half a
millisecond after sufficient current flows through a bridge wire in
each cap, e.g. DuPont "SSS" seismograph jet tapper electric blasting
caps. Energy through firing lead 52 is delivered to each cap 50 at
the same time so that all caps 50 detonate simultaneously to
generate the maximum explosion within envelope 13.
FIG. 9 discloses still another means for detonating the mixture in
envelope 13 which can be used in combination with any of the
detonation means disclosed above. Metallic dust, flakes, small bits
of wire, or the like 55, e.g. aluminum or organic dust, e.g. grain
dust, is sprayed or otherwise distributed into the methane-oxygen
mixture in envelope 13.
One way of distributing this material is
shown in FIG. 9 wherein the dust particles are sprayed out under
pressure through inlet conduit 56. Outlet conduit 57, continuously
sucks a portion of the dust and mixture from envelope 13 and
circulates some through mixing blower 58 and back into envelope 13
through inlet conduit 56. For the above example, it is estimated
that a 200-h.p. blower 58 will completely recirculate the contents
of envelope 13 every hour.
Aluminum dust concentrations as low 0.025 ounce per cubic foot in
air are known to explode violently. The dust may be ignited by a
modest radio signal. If the aluminum dust is ignited by a remote
radar signal, it would take the radar energy 0.3 microseconds to
travel the diameter of a 268 foot sphere.
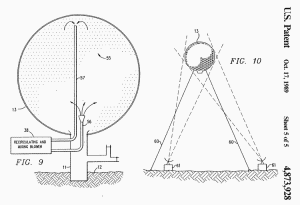
Fig 9-10
It is estimated that the methane-oxygen
detonation wave travels 6000 feet per second so where the aluminum
dust particles are separate by 254 microns, the explosion within
envelope 13 will take about 0.2 microseconds after the aluminum is
ignited. This results in the entire methane-oxygen explosion taking
place in less than one microsecond, making it as fast or faster than
an equivalent T.N.T. explosion or nuclear explosion.
As stated above, dust particles can be dispersed into the envelope
13 and used in conjunction with any of the detonating means
disclosed in FIGS. 2 through 8. In every instance the dust particles
will enhance the explosion of the methane-oxygen mixture.
FIG. 10 discloses a modification of the present invention wherein
the explosion is to be carried out above the earth's surface.
Envelope 13 is first partially filled with a non-explosive
methane-oxygen mixture as described above and then topped-out with
the addition oxygen just prior to launch. The gas mixture within
envelope 13 provides more than sufficient buoyancy for lifting the
filled envelope 13 to an altitude above the earth's surface.
Envelope 13 may be tethered in position by one or more tether lines
60 and is detonated by a firing lead within lines 60 or by radar or
radio frequency energy transmitted by one or more antennae 61.
While methane is considered to be the preferably combustible gas in
the present invention, there may be instances where other gas or
combinations of gases may be considered. For example, hydrogen
yields substantially less Btus per unit volume than methane when
exploded but has a much lower mass per molecule. Since the speed of
detonation increases as the mass of the molecules of the combustible
gas decreases, the continuity of the detonation front through the
explosive mixture should be improved by substituting an amount of
hydrogen, e.g. 50% by volume, for a like volume of methane within
envelope 13.
Also, while the gas-containing structure has been described as an
inflatable, spherical envelope, it could take other shapes and
configurations without departing from the present invention. For
example, other inflatable or expandable shapes can be used such as
dirigible-shaped envelopes, cylindrical envelopes, irregular-shaped
envelopes, etc.
Further, the structure may be an
inflatable envelope in connection with a rigid framework, e.g.
geodesic domes; a rigid base structure with only an inflatable
covering or roof, e.g. domed stadium-like structures; or fully
rigid, expendable structures, e.g. greenhouse-like structures.
The primary consideration is to loosely
contain the desired volume of explosive gas mixture so that when
detonated, an explosion having the desired yield will result.
|