
by Michio Kaku
Issue 107 / February 2005
from
ProspectMagazine Website
recovered from
TheHomesteadArchives Website
The universe is destined
to end. Before it does, could an advanced civilization
escape via a "wormhole" into a parallel universe? The
idea seems like science fiction, but it is consistent
with the laws of physics and biology. Here's how to do
it
The author is professor of theoretical physics at City
University of New York. This article is adapted from his
book "Parallel Worlds" (Allen Lane) |
The universe is out of control, in a runaway acceleration.
Eventually all intelligent life will face the final doom ”the big
freeze." An advanced civilization must embark on the ultimate
journey: fleeing to a parallel universe.
In Norse mythology,
Ragnarok ”the fate of the gods” begins
when the earth is caught in the vice-like grip of a bone-chilling
freeze. The heavens themselves freeze over, as the gods perish in
great battles with evil serpents and murderous wolves. Eternal
darkness settles over the bleak, frozen land as the sun and moon are
both devoured. Odin, the father of all gods, finally falls to his
death, and time itself comes to a halt.
Does this ancient tale foretell our future?
Ever since the work of Edwin Hubble
in the 1920s, scientists have known that the universe is expanding,
but most have believed that the expansion was slowing as the
universe aged. In 1998, astronomers at the Lawrence Berkeley
National Laboratory and the Australian National University
calculated the expansion rate by studying dozens of powerful
supernova explosions within distant galaxies, which can light up the
entire universe. They could not believe their own data. Some unknown
force was pushing the galaxies apart, causing the expansion of the
universe to accelerate.
Brian Schmidt, one of the group
leaders, said,
“I was still shaking my head, but we
had checked everything! I was very reluctant to tell people,
because I truly thought that we were going to get massacred.”
Physicists went scrambling back to their
blackboards and realized that some “dark energy” of unknown origin,
akin to Einstein’s “cosmological constant,” was acting as an
anti-gravity force. Apparently, empty space itself contains enough
repulsive dark energy to blow the universe apart.
The more the
universe expands, the more dark energy there is to make it expand
even faster, leading to an exponential runaway mode.
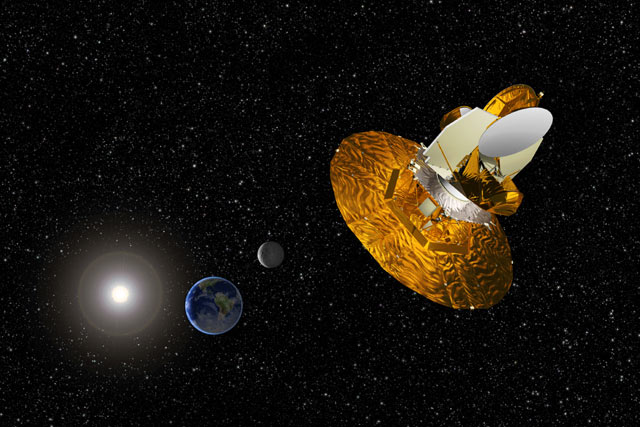
The WMAP space craft.
Image courtesy the
NASA/WMAP Science TEAM
In 2003, this astonishing result was confirmed by the WMAP (Wilkinson
microwave anisotropy probe) satellite.
Orbiting at a million
miles from earth, this satellite contains two telescopes capable of
detecting the faint microwave radiation which bathes the universe.
It is so sensitive that it is able to photograph in exquisite detail
the afterglow of the microwave radiation left over from the big
bang, which is still circulating the universe.
The WMAP satellite,
in effect, gave us “baby pictures” of the universe when it was a
mere 380,000 years old.
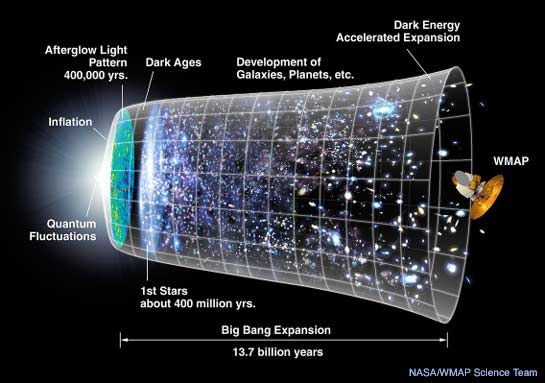
Timeline of the
universe: The expansion of the universe over most of it's history
has been relatively gradual.
The notion that a
rapid period "inflation" preceded the Big Bang expansion was first
put forth 25 years ago.
The new WMAP
observations favor specific inflation scenarios over other long held
ideas.
Credit: NASA
The WMAP satellite settled the long-standing question of the age of
the universe: it is officially 13.7bn years old (to within 1 per
cent accuracy).
But more remarkably, the data showed that dark
energy is not a fluke, but makes up 73 per cent of the matter and
energy of the entire universe. To deepen the mystery, the data
showed that 23 per cent of the universe consists of “dark matter,” a
bizarre form of matter which is invisible but still has weight.
Hydrogen and helium make up 4 per cent,
while the higher elements, you and I included, make up just 0.03 per
cent. Dark energy and most of dark matter do not consist of atoms,
which means that, contrary to what the ancient Greeks believed and
what is taught in every chemistry course, most of the universe is
not made of atoms at all.
As the universe expands, its energy content is diluted and
temperatures eventually plunge to near absolute zero, where even
atoms stop moving. One of the iron laws of physics is the second law
of thermodynamics, which states that in the end everything runs
down, that the total “entropy” (disorder or chaos) in the universe
always increases. This means that iron rusts, our bodies age and
crumble, empires fall, stars exhaust their nuclear fuel, and the
universe itself will run down, as temperatures drop uniformly to
near zero.
Charles Darwin was referring to this law when he wrote:
“Believing as I do that man in the
distant future will be a far more perfect creature than he now
is, it is an intolerable thought that he and all other sentient
beings are doomed to complete annihilation after such
long-continued slow progress.”
And one of the most depressing passages
in the English language was written by Bertrand Russell, who
described the “unyielding despair” he felt when contemplating the
distant future:
“No fire, no heroism, no intensity
of thought or feeling, can preserve a life beyond the grave; all
the labours of the ages, all the devotion, all the inspiration,
all the noonday brightness of human genius, are destined to
extinction in the vast death of the solar system; and the whole
temple of man’s achievement must inevitably be buried beneath
the debris of a universe in ruins.”
Russell wrote this passage in an era
before space travel, so the death of the sun does not seem so
catastrophic today ”but the death of the entire universe seems
inescapable." So on some day in the far future, the last star will
cease to shine, and the universe will be littered with nuclear
debris, dead neutron stars and black holes.
Intelligent civilizations, like homeless
people in rags huddled next to dying campfires, will gather around
the last flickering embers of black holes emitting a faint
Hawking
radiation.
String theory
to the rescue?
Although thermodynamics and cosmology point to the eventual death of
all life-forms in the universe, there is still one loophole. It is a
law of evolution that, when the environment changes radically, life
must adapt, flee or die. The first alternative seems impossible. The
last is undesirable. This leaves us with one choice: leave the
universe.
Although the concept of leaving our dying universe to enter another
seems utterly mad, there is no law of physics forbidding entering a
parallel universe. Einstein’s general relativity theory allows for
the existence of “wormholes” or gateways connecting parallel
universes, sometimes called “Einstein-Rosen bridges.” But it is
still unknown whether quantum corrections make such a journey
possible or not.
Although once considered a preposterous idea, the concept of the “multiverse”
- that our universe coexists with an infinite number of parallel
universes - has recently generated much interest among physicists
from several directions. First, the leading theory consistent with
the WMAP data is the “inflationary” theory, proposed by Alan Guth
of MIT in 1979. It postulates a turbo-charged expansion of the
universe at the beginning of time. The
inflationary universe idea
neatly explains several stubborn cosmological mysteries, including
the flatness and uniformity of the universe.
But since physicists still do not know what drove this rapid
inflationary process, there remains the chance that it could happen
again, in an endless cycle. This is the chaotic inflationary idea of
Andrei Linde of Stanford University, in which “parent
universes” bud “baby universes” in a continuous, never-ending cycle.
Like soap bubbles which split into two smaller bubbles, universes
can constantly sprout from other universes.
But what caused the big bang and drove this inflation?
The question remains unanswered. Since
the big bang was so intense, we have to abandon Einstein’s theory of
general relativity, which forms the underlying framework for all of
cosmology. Einstein’s theory of gravity breaks down at the instant
of the big bang, and hence cannot answer the deep philosophical and
theological questions raised by this event. At these incredible
temperatures, we must incorporate quantum theory ”the other great
theory to emerge in the 20th century” which governs the physics of
the atom.
Quantum theory and Einstein’s relativity theory are opposites.
The
former governs the world of the very small, the peculiar subatomic
realm of electrons and quarks. Relativity theory rules the world of
the very large of black holes and expanding universes. Relativity,
therefore, is not suited to explaining the instant of the big bang,
where the universe was smaller than a subatomic particle. At this
moment we would expect radiation effects to dominate over gravity,
and hence we need a quantum description of gravity. Indeed, one of
the greatest challenges facing physics is to unify these theories
into a single, coherent theory of all the forces in the universe.
Physicists today are groping for this “theory of everything.” Many
proposals have been made over the past half century, but all have
been shown to be inconsistent or incomplete. So far, the leading, in
fact the only ”candidate", is
string theory.
The latest incarnation of string theory,
M-theory, may answer a
question which has dogged advocates of higher dimensions for a
century: where are they?
Smoke can expand and fill up an entire room
without vanishing into hyperspace, so higher dimensions, if they
exist at all, must be smaller than an atom. If higher-dimensional
space were larger than an atom, then we should see atoms
mysteriously drifting and disappearing into a higher dimension,
which we do not see in the laboratory.
In the older string picture, one had to “curl” or wrap up six of the
ten original dimensions, leaving the four-dimensional universe of
today. These unwanted dimensions were squeezed into a tiny ball
(called a
Calabi-Yau manifold) too small to be seen. But M-theory
adds a new twist to this: some of these higher dimensions can be
large, or even infinite, in size.
Imagine two parallel sheets of paper. If
an ant lived on each sheet, each would think that its sheet was the
entire universe, unaware that there was another universe close by.
In fact, the other universe would be invisible. Each ant would live
out its life oblivious to the fact that another universe was only a
few inches away. Similarly, our universe may be a membrane floating
in 11-dimensional hyperspace, while we remain oblivious of the
parallel universes hovering nearby.
One interesting version of M-theory cosmology is the “ekpyrotic”
(from the Greek for “conflagration”) universe, proposed by Paul
Steinhardt, Burt Ovrut and Neil Turok. It assumes
that our universe is a flat, infinite membrane floating in
higher-dimensional space. But occasionally, gravity attracts a
nearby membrane. These two parallel universes race towards each
other until they collide, releasing a colossal amount of energy (the
big splat).
This explosion creates our known
universe and sends the two parallel universes flying apart in
hyperspace.
Searching for
higher dimensions
The intense interest in higher dimensions generated by string theory
has slowly spilled over into the world of experimental physics. Idle
dinner-table chatter is being translated into multimillion-dollar
physics experiments.
At the University of Colorado in Denver, the first experiment was
conducted to search for the presence of a parallel universe, perhaps
only a millimeter away. Physicists searched for tiny deviations from
Newton’s inverse square law for gravity. The light from a candle is
diluted as it spreads out, decreasing at the inverse square of the
distance of separation. Similarly, according to Newton’s law,
gravity also spreads out over space and decreases in the same way.
But in a four-dimensional universe, there is more room for light or
gravity to spread out, so they decrease at the inverse cube of the
distance. Hence, by searching for tiny deviations from the inverse
square law, one may pick up the presence of the fourth dimension.
Newton’s inverse square law is so precise that it can guide our
space probes throughout the solar system. But no one knows if it
holds down to the millimeter level. At present, only null results
have been found in these experiments. Other groups are searching for
even smaller deviations. Physicists at Purdue University in Indiana
are trying to test the law down to the atomic level, using
nanotechnology.
Other avenues are also being explored. In 2007, the large hadron
collider (LHC), capable of blasting subatomic particles
with a colossal energy of 14 trillion electron volts (10 trillion
times the energy found in a typical chemical reaction) will be
turned on outside Geneva. The world’s largest atom smasher,
this huge machine, 27km in circumference, straddling the
French-Swiss border, will probe into places 10,000 times smaller
than a proton. Physicists expect to find an entire zoo of new
subatomic particles not seen since the big bang.
Physicists predict that the LHC may create exotic particles like
mini-black holes and supersymmetric particles, dubbed “sparticles,”
which would provide indirect evidence for string theory. In string
theory, every particle has a super-partner. The partner of the
electron is the “selectron,” the partner of the quark is the “squark,”
and so on.
Furthermore, around 2012, the space-based gravity wave detector
Lisa (laser interferometer space antenna) will be sent
into orbit. Lisa will be able to detect the gravitational shockwaves
emitted less than a trillionth of a second after the big bang. It
will consist of three satellites circling the sun, connected by
laser beams, making a huge triangle in space 5m km on each side. Any
gravitational wave which strikes Lisa will disturb the lasers, and
this tiny distortion will be picked up by instruments, signaling the
collision of two black holes or the big bang aftershock itself.
Lisa is so sensitive ”it can measure
distortions a tenth the diameter of an atom” that it may be able to
test many of the scenarios being proposed for the pre-big bang
universe, including string theory.
Steps to leave
the universe
Unfortunately, the energy necessary to manipulate these higher
dimensions, rather than just observe them, is far beyond anything
available to us in the foreseeable future: 1019 bn
electron volts, or a quadrillion times the energy of the large
hadron collider. To operate here one needs the technology of a
super-advanced civilization.
In order to organize a discussion of advanced extraterrestrial
civilizations, astrophysicists often use the classification of
Type
I, II and III civilizations introduced by Russian astrophysicist
Nikolai Kardashev in the 1960s, who ranked them by their energy
consumption.
One might expect that a Type III civilization, using the full
power of its unimaginably vast galactic resources, would be able to
evade the big freeze. The bodies of its citizens, for example, might
be genetically altered and their organs replaced by computerized
implants, representing a sophisticated merger of silicon and carbon
technologies. But even these superhuman bodies would not survive the
big freeze. This is because we define intelligence as the ability to
process information.
According to physics, all machines,
whether they are computers, rockets, locomotives or steam engines,
ultimately depend on extracting energy from temperature differences:
steam engines, for example, work by extracting energy from boiling
water. But information-processing, and hence intelligence, requires
energy supplied by machines and motors, which will become impossible
as temperature differences drop to zero. According to the laws of
physics, in a uniformly cold universe where temperature differences
do not exist, intelligence cannot survive.
But since the big freeze is probably billions to trillions of years
away, there is time for a Type III civilization to plot the only
strategy consistent with the laws of physics: leaving this universe.
To do this, an advanced civilization will first have to discover the
laws of quantum gravity, which may or may not turn out to be string
theory. These laws will be crucial in calculating several unknown
factors, such as the stability of wormholes connecting us to a
parallel universe, and how we will know what these parallel worlds
will look like.
Before leaping into the unknown, we have
to know what is on the other side. But how do we make the leap?
Here are some of the ways.
Find a naturally occurring wormhole
An advanced civilization which has
colonized the galaxy may have stumbled during its past
explorations upon exotic, primordial left-overs from the big
bang. The original expansion was so rapid and explosive that
even tiny wormholes might have been stretched and blown up into
macroscopic size. Wormholes, cosmic strings, negative matter,
negative energy, false vacua and other exotic creatures of
physics may be relics left over from creation.
But if such naturally occurring gateways are not found, then the
civilization will have to take more complex and demanding steps.
Send a probe through a black hole
Black holes, we now realize, are
plentiful; there is one lurking in the centre of our own milky
way galaxy weighing about 3m solar masses. Probes sent through a
black hole may settle some unsolved questions. In 1963, the
mathematician Roy Kerr showed that a rapidly spinning black hole
will not collapse into a dot, but rather into a rotating ring,
which is kept from collapsing by centrifugal forces.
All black holes are surrounded by an event horizon, or point of
no return: passing through the event horizon is a one-way trip.
Conceivably, two such black holes would be needed for a return
trip. But to an advanced civilization fleeing the big freeze, a
one-way trip may be all that is required.
What happens if one falls through the Kerr ring is a matter for
debate. Some believe that the act of entering the wormhole will
close it, making it unstable. And light falling into the black
hole would be blue-shifted, giving rise to the possibility that
one might be fried as one passed into a parallel universe. No
one knows for sure, so experiments must be done.
This controversy heated up last year
when Stephen Hawking admitted that he had made a mistake
30 years ago in betting that black holes gobble up everything,
including information. Perhaps the information is crushed
forever by the black hole, or perhaps it passes into the
parallel universe on the other side of the Kerr ring. Hawking’s
latest thinking is that information is not totally lost. But no
one believes that the final word on this delicate question has
been spoken.
To gain further data on space-times which are stretched to
breaking point, an advanced civilization might create a black
hole in slow motion. In 1939, Einstein analyzed a rotating mass
of stellar debris which was slowly collapsing under its own
gravity. Although Einstein showed that this rotating mass would
not collapse into a black hole, an advanced civilization may
duplicate this experiment in slow motion by collecting a
swirling mass of neutron stars weighing less than about 3 solar
masses and then gradually injecting extra stellar material into
the mass, forcing it to undergo gravitational collapse.
Instead of collapsing into a dot, it
will collapse into a ring, and hence allow scientists to witness
the formation of a Kerr black hole in slow motion.
Create negative energy
If Kerr rings prove to be
too unstable or lethal, one might also contemplate opening up
wormholes via negative matter/energy. In 1988, Kip Thorne and
his colleagues at the California Institute of Technology showed
that if one had enough negative matter or negative energy, one
could use it to create a "transversable wormhole” one in which
you could pass freely back and forth between your lab and a
distant point in space (and even time). Negative matter/energy
would be sufficient to keep the throat of the wormhole open for
travel.
Unfortunately, no one has ever seen negative matter. In
principle, it should weigh less than nothing and fall up, rather
than down. If it existed when the earth was created, it would
have been repelled by the earth’s gravity and drifted off into
space.
Negative energy, however, has been seen in the laboratory in the
form of
the Casimir effect. Normally, the force between two
uncharged parallel plates should be zero. But if quantum
fluctuations outside the plates are greater than the
fluctuations between the plates, a net compression force will be
created. The fluctuations pushing the plates from the outside
are larger than the fluctuations pushing out from within the
plates, so these uncharged plates are attracted to each other.
This was first predicted in 1948 and measured in 1958. However,
the Casimir energy is tiny proportional to the inverse fourth
power of the separation of the plates. To make use of the
Casimir effect would require advanced technology to squeeze
these parallel plates to very small separations. If one were to
reshape these parallel plates into a sphere with a double
lining, and use vast amounts of energy to press these spherical
plates together, enough negative energy might be generated for
the interior of the sphere to separate from the rest of the
universe.
Another source of negative energy is laser beams. Pulses of
laser energy contain “squeezed states,” which contain negative
as well as positive energy. The problem is separating the
negative from the positive energy within the beam. Although this
is theoretically possible, it is exceedingly difficult. If a
sophisticated civilization could do this, then powerful laser
beams might generate enough negative energy for the sphere to
peel from our universe.
Even black holes have negative energy surrounding them, near
their event horizons. In principle, this may yield vast
quantities of negative energy. However, the technical problems
of extracting negative energy so close to a black hole are
extremely tricky.
Create a baby universe
According to inflation,
just a few ounces of matter might suffice to create a baby
universe. This is because the positive energy of matter cancels
out the negative energy of gravity. If the universe is closed,
then they cancel out exactly. In some sense, the universe may be
a free lunch, as Guth has emphasized. Strange as it may seem, it
requires no net energy to create an entire universe.
Baby universes are in principle
created naturally when a certain region of space-time becomes
unstable and enters a state called the “false vacuum,” which
destabilizes the fabric of space-time. An advanced civilization
might do this deliberately by concentrating energy in a single
region. This would require either compressing matter to a
density of 1080 g/cm3, or heating it to 1029
degrees kelvin.
To create the fantastic conditions necessary to open up a
wormhole with negative energy or to create a false vacuum with
positive energy, one might need a “cosmic atom-smasher.”
Physicists are attempting to build “table-top” accelerators that
can, in principle, attain billions of electron volts on a
kitchen table. They have used powerful laser beams to attain an
energy acceleration of 200bn electron volts per meter, a new
record. Progress is rapid, with the energy growing by a factor
of ten every five years. Although technical problems still
prevent a true table-top accelerator, an advanced civilization
has billions of years to perfect these and other devices.
To reach the
Planck energy (1028 eV) with this laser
technology would require an atom-smasher ten light years long,
beyond the nearest star, which would be well within the
technological capabilities of a Type III civilization. Since the
vacuum of empty space is better than any vacuum attainable on
the earth, the beam of subatomic particles may not need light
years of tubing to contain it; it could be fired in empty space.
Power stations would have to be placed along the path in order
to pump laser energy into the beam, and also to focus it.
Another possibility would be to bend the path into a circle so
that it fits within the solar system. Gigantic magnets could be
placed on asteroids to bend and focus the beam in a circular
path around the sun. The magnetic field necessary to bend the
beam would be so huge that the surge of power through the coils
might melt them, meaning that they could only be used once.
After the beam had passed, the
melted coils would have to be discarded and replaced in time for
the next pass.
Build a laser implosion machine
In principle, it might be possible to create laser beams of
limitless power; the only constraints are the stability of the
lasing material and the energy of the power source. In the lab,
terawatt (trillion watt) lasers are now common, and petawatt
(quadrillion watt) lasers are slowly becoming possible (in
comparison, a commercial nuclear power plant generates only a
billion watts of continuous power).
One can even envisage an X-ray laser
powered by the output of a hydrogen bomb, which would carry
unimaginable power in its beam. At the Lawrence Livermore
National Laboratory, a battery of lasers is fired radially on a
small pellet of lithium deuteride, the active ingredient of a
hydrogen bomb, in order to tame the power of thermonuclear
fusion.
An advanced civilization might create huge laser stations on the
asteroids and then fire millions of laser beams on to a single
point, creating vast temperatures and pressures unimaginable
today.
Send a nanobot to recreate
civilization
If the wormholes created in the previous steps are too small,
too unstable, or the radiation effects too intense, then perhaps
we could send only atom-sized particles through a wormhole. In
this case, this civilization may embark upon the ultimate
solution: passing an atomic-sized “seed” through the wormhole
capable of regenerating the civilization on the other side. This
process is commonly found in nature.
The seed of an oak tree, for
example, is compact, rugged and designed to survive a long
journey and live off the land. It also contains all the genetic
information needed to regenerate the tree.
An advanced civilization might want to send enough information
through the wormhole to create a “nanobot,” a self-replicating
atomic-sized machine, built with nanotechnology. It would be
able to travel at near the speed of light because it would be
only the size of a molecule. It would land on a barren moon, and
then use the raw materials to create a chemical factory which
could create millions of copies of itself.
A horde of these robots would then
travel to other moons in other solar systems and create new
chemical factories. This whole process would be repeated over
and over again, making millions upon millions of copies of the
original robot. Starting from a single robot, there will be a
sphere of trillions of such robot probes expanding at near the
speed of light, colonizing the entire galaxy.
(This was the basis of
the movie 2001, probably the most
scientifically accurate fictional depiction of an encounter with
an extraterrestrial life-form. Instead of meeting aliens in a
flying saucer or the USS Enterprise, the most realistic
possibility is that we will make contact with a robot probe left
on a moon from a passing Type III civilization. This was
outlined by scientists in the opening minutes of the film, but
Stanley Kubrick cut the interviews from the final edit.)
Next, these robot probes would create huge biotechnology
laboratories. The DNA sequences of the probes’ creators would
have been carefully recorded, and the robots would have been
designed to inject this information into incubators, which would
then clone the entire species. An advanced civilization may also
code the personalities and memories of its inhabitants and
inject this into the clones, enabling the entire race to be
reincarnated.
Although seemingly fantastic, this scenario is consistent with
the known laws of physics and biology, and
is within the
capabilities of a Type III civilization. There is nothing in the
rules of science to prevent the regeneration of an advanced
civilization from the molecular level.
For a dying civilization trapped in a
freezing universe, this may be the last hope.
|