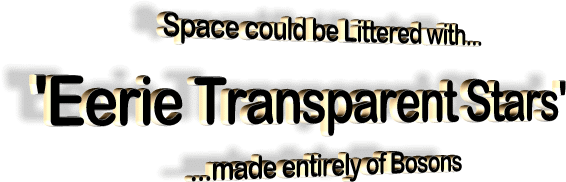
by Michelle Starr
September
09, 2020
from
ScienceAlert Website
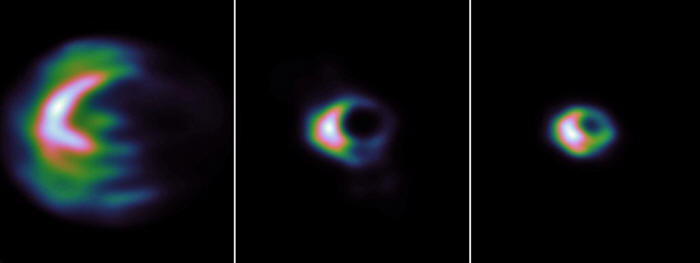
L-r: A non-rotating black hole;
a
rotating black hole;
a
boson star as they'd appear to
the Event Horizon Telescope (EHT).
(Olivares et al., MNRAS, 2020)
Last year, the astronomical community achieved an absolute wonder.
For the very first time,
the world collectively laid eyes on an
actual image of the shadow of
a black hole. It was the culmination of years of work, a magnificent
achievement in both human collaboration and technical ingenuity.
And, like the best scientific breakthroughs, it opened a whole new
world of enquiry.
For a team led by astrophysicist
Hector Olivares
from Radboud University in the Netherlands and Goethe University in
Germany, that enquiry was:
how do we know
M87*
is a
black hole?
"While the image is
consistent with our expectations on what a black hole would look
like, it is important to be sure that what we are seeing is
really what we think," Olivares told ScienceAlert.
"Similarly to black holes, boson stars are predicted by general
relativity and are able to grow to millions of solar masses and
reach a very high compactness.
The fact that they
share these features with supermassive black holes led
some
authors to propose that some of the
supermassive compact objects
located at the
center of galaxies could actually be
boson
stars."
So, in a new paper,
Olivares and his team have calculated what a boson star might look
like to one of our telescopes, and how that would differ from a
direct image of an accreting black hole.
Boson stars are among the strangest theoretical objects out there.
They're not much like conventional stars, except that they're a glob
of matter. But where stars are primarily made up of particles called
fermions - protons, neutrons, electrons, the stuff that forms more
substantial parts of our Universe - boson stars would be made up
entirely of... bosons.
These particles - including photons, gluons and the famous
Higgs
boson - don't follow the same physical rules as
fermions.
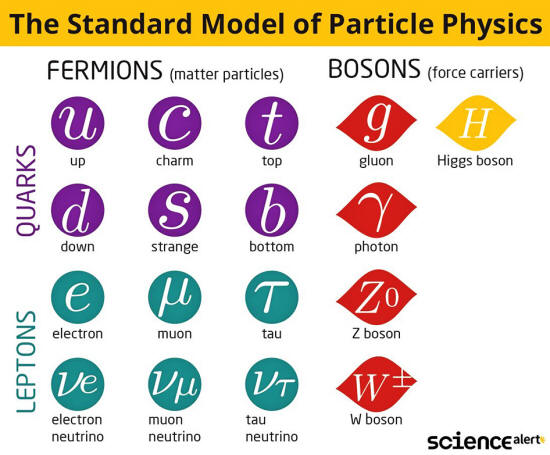
Chart of fermions and bosons
Fermions are subject to the
Pauli exclusion principle, which means
you can't have two identical particles occupying the same space.
Bosons, however, can be
superimposed:
when they come together, they act like one big
particle or
matter wave.
We know this, because it's been done in a
lab, producing what we call a
Bose-Einstein condensate.
In the case of boson stars, the particles can be squeezed into a
space which can be described with distinct values, or points on a
scale. Given the right kind of bosons in the right arrangements,
this 'scalar field' could fall into a relatively stable arrangement.
That's the theory, at least...
Not that anybody has seen one in
action. Bosons with the mass required to form such a structure, let
alone one with the mass of a supermassive black hole, are yet to be
spotted.
If we could identify a boson star, we would have effectively located
this elusive particle.
"In order to form a
structure as large as the
SMBH candidates, the mass of the boson
needs to be extremely small (less than 10-17 electronvolts),"
Olivares said.
"Spin-0 bosons with similar or smaller masses appear in several
cosmological models and
string theories, and have been proposed
as dark matter candidates under different names:
-
scalar field
dark matter
-
ultra-light axions
-
fuzzy dark matter
-
quantum wave
dark matter
Such hypothetical
particles would be extremely difficult to detect, but the
observation of an object looking like a boson star would point
to their existence."
Boson stars do not fuse
nuclei, and they would not emit any radiation.
They'd just sit there
in space, being invisible. Much like black holes...
Unlike black holes, however, boson stars would be
transparent - they
lack an absorbing surface that would stop photons, nor do they have
an event horizon.
Photons can escape boson
stars, although their path may be
bent a little by the gravity.
But some boson stars may be surrounded by a rotating ring of plasma
- a lot like the accretion disc that surrounds a black hole. And it
would look fairly similar, like a glowing doughnut with a dark
region inside.
So, Olivares and his team performed simulations of the dynamics of
these plasma rings, and compared them to what we might expect to see
of a black hole.
"The plasma
configuration that we use is not set up 'by hand' (under
reasonable assumptions), but results from a simulation of plasma
dynamics.
This allows the plasma to evolve in time and to form
structures as it would in nature," Olivares explained.
"In this way we could relate the size of the dark region in the
boson star images (which mimics a black hole shadow) to the
radius where a plasma instability stops operating.
In turn, this means
that the size of the dark region is not arbitrary - it will
depend on the properties of the boson star space-time - and also
allows us to predict its size for other boson stars that we have
not simulated."
They found that the boson
star's shadow would be significantly smaller than the shadow of a
black hole of similar mass.
Thus,
M87* could be ruled
out as a boson star - at least as modeled by the team.
"The mass of [M87*]
inferred from stellar dynamics is consistent with the
expectations on the size of its shadow for the case of a black
hole, so the dark region is too big to correspond to a
non-rotating boson star similar to those we studied," Olivares
told ScienceAlert.
But the team also took
into account the technical capabilities and limitations of the
Event
Horizon Telescope (EHT) which delivered that first black hole image:
they
deliberately set about visualizing their results as they thought
boson stars might look as imaged by the EHT.
This means their results can be compared to future EHT observations,
to determine if what we're looking at is indeed a supermassive black
hole.
If it were not, that would be a very big deal. It wouldn't mean that
supermassive black holes don't exist - the range of masses for black
holes is way too broad for boson stars.
But it would hint that
boson stars are real, and in turn that would have huge implications,
for everything from the
inflation of the early Universe to the
search for
dark matter.
"It would mean that
cosmological scalar fields exist and play an important role in
the formation of structures in the Universe," Olivares told
ScienceAlert.
"The growth of supermassive black holes is still not understood
very well, and if it turns out that at least some of the
candidates are actually boson stars, we would need to think of
different formation mechanisms involving scalar fields."
The
research was
published in July in the Monthly Notices of the Royal Astronomical
Society...
|