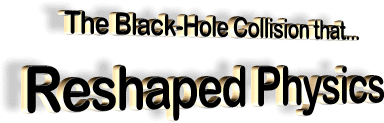
by Davide Castelvecchi
23 March 2016
from
Nature Website
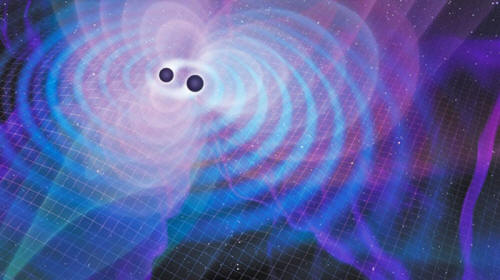
Binary black holes
radiate a huge amount
of orbital energy as
gravitational waves.
Illustration by Mark
Garlick
A momentous signal from space
has confirmed decades of theorizing on
black holes
- and launched a new era
of gravitational-wave
astronomy.
The event was catastrophic on a cosmic
scale - a merger of black holes that violently shook the surrounding
fabric of space and time, and sent a blast of
space-time vibrations known as gravitational waves (below video) rippling
across the Universe at the speed of light.
But it was the kind of calamity that
physicists on Earth had been waiting for.
On 14 September, when
those ripples swept across the freshly upgraded
Laser Interferometer Gravitational-Wave Observatory (Advanced
LIGO), they showed up as spikes in the readings from its two
L-shaped detectors in Louisiana and Washington state.
For the first
time ever,
scientists had recorded a gravitational-wave signal.
"There it was!" says LIGO team member
Daniel Holz, an astrophysicist at the University of Chicago in
Illinois. "And it was so strong, and so beautiful, in both
detectors."
Although the shape of the signal looked familiar from
the theory, Daniel Holz says,
"it's completely different when you see
something in the data. It's this transcendent moment".
The signal, formally designated GW150914
after the date of its occurrence and informally known to its
discoverers as ''the Event'', has justly been hailed as a milestone in
physics.
It has provided a wealth of evidence for
Albert Einstein's century-old general theory of relativity,
which holds that mass and energy can warp space-time, and that
gravity is the result of such warping.
Stuart Shapiro, a specialist
in computer simulations of relativity at the University of Illinois
at Urbana-Champaign, calls it,
"the most significant confirmation of
the general theory of relativity since its inception".
But 'the Event' also marks the start of a
long-promised era of gravitational-wave astronomy.
Detailed analysis
of the signal has already yielded insights into the nature of the
black holes that merged, and how they formed. With more events such
as these - the LIGO team is analyzing several other candidate events
captured during the detectors' four-month run, which ended in
January - researchers will be able to classify and understand the
origins of black holes, just as they are doing with stars.
Still more events should appear starting
in September, when Advanced LIGO is scheduled to begin joint
observations with its European counterpart, the Franco-Italian-led
Advanced Virgo facility near Pisa, Italy.
(The two collaborations
already pool data and publish papers together.)
This detector will
not only contribute crucial details to events, but could also help
astronomers to make cosmological-distance measurements more
accurately than before.
"It's going to be a really good ride for
the next few years," says Bruce Allen, managing director of the Max
Planck Institute for Gravitational Physics in Hanover, Germany.
"The more black holes they see whacking
into each other, the more fun it will be," says Roger Penrose, a
theoretical physicist and mathematician at the University of Oxford,
UK, whose work in the 1960s helped to lay the foundation for the
theory of the objects.
"Suddenly, we have a new way of
looking at the Universe."
A matter of energy
Physicists have known for decades that
every pair of orbiting bodies is a source of gravitational waves.
With each revolution, according to Einstein's equations, the waves
will carry away a tiny fraction of their orbital energy. This will
cause the objects to move a bit closer together and orbit a little
faster.
For familiar pairs, such as the Moon and Earth, such energy
loss is imperceptible even on timescales of billions of years. But dense objects in very close orbits
can lose energy much more quickly.
In 1974, radio astronomers
Russell Hulse and Joseph Taylor, then of the University of
Massachusetts Amherst,
found just such a system:
a pair of dense neutron stars in orbit
around each other.
As the years went by, the scientists found that
this 'binary pulsar' was losing energy and spiraling inwards
exactly as predicted by Einstein's theory.
The two black holes detected by LIGO had
probably been losing energy in this way for millions, if not
billions, of years before they reached the end.
But LIGO did not
register the gravitational waves coming from them until 9:50:45
Coordinated Universal Time on 14 September, when the wave's
frequency rose above some 30 cycles per second (hertz) - corresponding to 15 full black-hole orbits per second
- and was
finally high enough for the detectors to distinguish it from
background noise.
But then, in just 0.2 seconds, LIGO
watched the signal surge to 250 hertz and suddenly disappear, as the
black holes made their final 5 orbits, reached orbital velocities of
half the speed of light and coalesced into a single massive object.
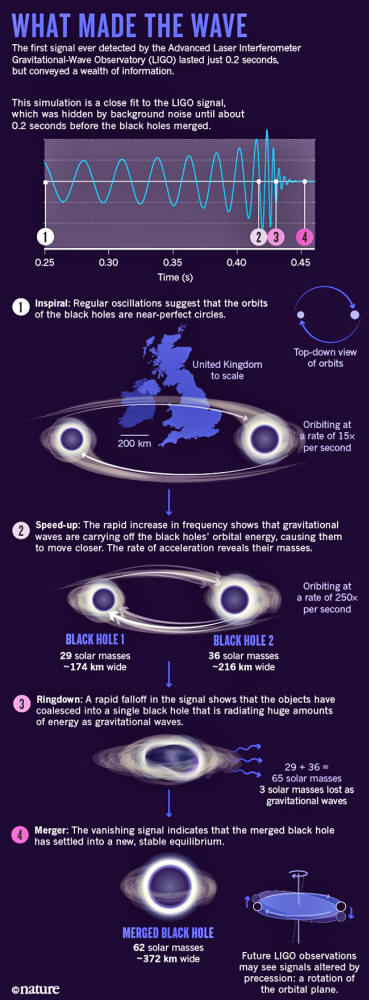
The LIGO and Virgo teams soon went
to work
extracting every bit of information possible.
At the most
fundamental level, the signal gave them an existence proof: the
fact that the objects came so close to each other before merging
meant that they had to be black holes, because ordinary stars
would need to be much bigger.
"It is, I think, the clearest
indication that black holes are really there," says Penrose.
The signal also provided researchers
with the first empirical test of general relativity beyond
regions - including the space around the binary pulsar - where
there is comparatively little space-time warping.
There was no
empirical evidence that the theory would keep its validity at
the extreme energies of merging black holes, says Shapiro - but
it did.
The signal held a trove of
more-detailed information as well. By scrutinizing its shape
just before the final cataclysm, the scientists found that it
closely approximated a simple sine wave with a steadily
increasing frequency and amplitude.
According to B.S. Sathyaprakash, a theoretical physicist at Cardiff University,
UK, and a senior LIGO researcher, this pattern suggests that the
orbits of the black holes were nearly circular, and that LIGO
probably had a bird's-eye view of the circles, looking almost
straight down on them rather than edge-on.
In addition, the LIGO and Virgo
teams were able to use the frequency of the observed wave, along
with its rate of acceleration, to estimate the masses of the two
black holes:
because heavier objects radiate energy in the form
of gravitational waves at a faster rate than do lighter objects,
their pitch rises more quickly.
By recreating 'the Event' with
computer simulations, the scientists calculated that the two
black holes weighed about 36 times and 29 times the mass of the
Sun, respectively, and that the combined black hole weighed
about 62 solar masses.
1
The lost difference, about three Suns' worth, was dispersed as
gravitational radiation - much of it during what physicists call
the 'ringdown' phase, when the merged black hole was settling
into a spherical shape.
(For comparison, the most powerful
thermonuclear bomb ever detonated converted only about 2
kilograms of matter into energy - roughly 1030 times
less.)
The teams also suspect that the final black hole was
spinning at perhaps 100 revolutions per second, although the
margin of error on that estimate is large.
The inferred masses of the two black
holes are also revealing. Each object was presumably the remnant
of a very massive star, with the larger star approaching 100
times the mass of the Sun and the smaller one a little less.
Thermonuclear reactions are known to convert hydrogen in the
cores of such stars into helium much faster than in lighter
stars, which leads them to collapse under their own weight only
a few million years after they are born.
The energy released by
this collapse causes an
explosion called a type II supernova, which leaves behind a
residual core that turns into a neutron star or, if it's massive
enough, a black hole.
Scientists say that type II
supernovae should not produce black holes much bigger than about
30 solar masses - and both black holes were at the high end of
that range.
This could mean that the system formed from
interstellar gas clouds that were richer in hydrogen and helium
than the ones typically found in our Galaxy, and that were
poorer in heavy elements - which astronomers call metals.
Astrophysicists have calculated that
stars formed from such low-metallicity clouds should have an
easier time forming massive black holes when they explode,
explains Gijs Nelemans, an astronomer at Radboud University
Nijmegen in the Netherlands and a member of the Advanced Virgo
collaboration.
That's because during a supernova explosion,
smaller atoms are less likely to be blown away by the blast.
Low-metallicity stars thus,
"lose less mass, so more of it goes
into the black hole, for the same initial mass", Nelemans says.
Two by two
But how did these two black holes
end up in a binary system?
In a paper 2
published at the same time as the one reporting the discovery,
the LIGO and Virgo teams described two commonly accepted
scenarios.
The simplest one is that two massive
stars were born as a binary-star system, forming from the same
interstellar gas cloud like a double-yolked egg, and orbiting
each other ever since. (Such binary stars are common in our
Galaxy; singletons such as the Sun are the exception, rather
than the rule.)
After a few million years, one of the stars
would have burned out and gone supernova, soon to be followed by
the other. The result would be a binary black hole.
The second scenario is that the
stars formed independently, but still inside the same dense
stellar cluster - perhaps one similar to the globular clusters
that orbit the Milky Way.
In such a cluster, massive stars would
sink towards the centre and, through complex interactions with
lighter stars, form binary systems, possibly long after their
transformation into black holes.
"It is, I think, the clearest
indication that black holes are really there."
Simulations made by Simon Portegies
Zwart, an astrophysicist at Leiden University in the
Netherlands, show 3
that massive stars are more likely to form in dense clusters,
where collisions and mergers are more common.
He also finds that
once a binary black-hole system forms, the complex dynamics of
the cluster's centre would probably kick the pair out at high
speed. The binary that Advanced LIGO detected may have wandered
away from any galaxy for billions of years before merging, he
says.
Although the LIGO and Virgo teams
were able to learn a lot from 'the Event', there is much more that
gravitational waves could teach them, even in the case of
black-hole mergers.
The detectors showed that immediately after
the black holes merged, the waves quickly died down as the
resulting black hole settled into a symmetrical shape.
This is
consistent with predictions made by theoretical physicist C.V. Vishveshwara in the early 1970s, a time when,
"gravitational waves and black
holes both belonged to the realm of mythology", he says.
"At that time, I had not
imagined that it would ever be verified," says Vishveshwara,
who is director emeritus of the Jawaharlal Nehru Planetarium
in Bangalore, India.
But LIGO saw only just over one
cycle of the Event's ringdown waves before the signal became
buried once more in the background noise - not yet enough data
to provide a rigorous test of Vishveshwara's predictions.
More-stringent tests will be
possible if and when LIGO detects black-hole mergers that are
larger than this one, or that occur closer to Earth than the
Event's estimated distance of 1.3 billion light years, and thus
give 'louder' waves that stay above the noise for longer.
Alessandra Buonanno, a LIGO theorist
and director of the Max Planck Institute for Gravitational
Physics in Potsdam-Golm, Germany, says that a more detailed
picture of the ringdown stage could reveal how fast the final
black hole rotates, as well as whether its formation gave it a
'natal kick', imparting a high velocity.
In addition, says Sathyaprakash,
"we are especially waiting for
systems that are much lighter, so they last longer".
Such events could include the
mergers of lighter binary black holes, of binary neutron stars
or of a black hole with a neutron star.
Each type would deliver its own
signature chirp, and could produce a signal that stays above
LIGO's threshold of sensitivity for several minutes or more.
"GW150914 is in some sense a
very vanilla system," says Chad Hanna, a LIGO member at
Pennsylvania State University in University Park. "It's
beautiful, of course, but it doesn't have all the crazy
things that one might expect."
Space artistry
One phenomenon that Sathyaprakash is
eager to observe is a 'precession' of the black holes' orbital
plane, meaning that their paths trace a kind of 3D rosette.
This
is a relativistic effect that has no counterpart in Newtonian
gravity, and it should produce a characteristic fluctuation in
the strength of the gravitational waves. But orbital precession
occurs only when two black holes have axes of rotation that
point in random directions, and it disappears when the axes are
both perpendicular to the orbital plane.
The occurrence of a
precession could provide clues to how the black holes formed.
It's hard to be sure about that
possibility because there are many uncertainties in simulating
supernovas. But astrophysicists suspect that parallel spins
generally signify that the original two stars were born together
out of the same whirling gas cloud.
Similarly, they think that
random spins result from black holes that formed separately and
later fell into orbit around each other. Once the observatories
find more mergers, they may be able to determine which type of
system occurs more frequently.
Although detecting more events will
help LIGO to do lots of science, its interferometers have
intrinsic limitations that make it necessary to work together
with a worldwide network of similar detectors that are now
coming online.
First, LIGO's two interferometers
are not enough for scientists to determine precisely where the
waves came from. The researchers can get some information by
comparing the signal's time of arrival at each detector: the
difference enables them to calculate the wave's direction
relative to an imaginary line drawn between the two.
But in the
case of ''the Event'', which recorded a difference of 6.9
milliseconds, their calculations limited the field of
possibilities merely to a wide strip of southern sky.
Had Virgo been online, the
scientists could have narrowed down the direction substantially
by comparing the waves' arrival times at three places.
With a
fourth interferometer (Japan is building an underground one
called
KAGRA, for Kamioka Gravitational-Wave Detector, and India
has its own LIGO in planning), their precision would improve
much more.
Knowing an event's direction will in
turn remove one of the biggest uncertainties in determining its
distance from Earth.
Waves that approach from a direction
exactly perpendicular to the detector - either from above or
from below, through Earth - will be recorded at their actual
amplitude, explains Fulvio Ricci, a physicist at the University
of Rome La Sapienza and the spokesperson for Virgo.
Waves that
come from elsewhere in the sky, however, will hit the detector
at an angle and produce a somewhat smaller signal, according to
a known formula.
There are even some blind spots, where a source
cannot be seen by a given detector at all.
Determining the direction will
therefore reveal the exact amplitude of the waves. By comparing
that figure with the waves' amplitude at the source, which the
researchers can derive from the shape of the signal, and by
knowing how the amplitude decreases with distance, which they
get from Einstein's theory, they can then calculate the distance
of the source to a much higher precision.
This situation is almost
unprecedented:
conventionally, astronomical distances need to be
estimated by looking at the brightness of known objects in
locations that range from the Solar System to distant galaxies.
But the measured brightness of those 'standard candles' can be
dimmed by stuff in between. Gravitational waves have no such
limitation.
Raising the alarm
There is another important reason
why scientists are eager to have precise estimates of the waves'
provenance.
The LIGO and Virgo teams have arranged to give
near-real-time alerts of intriguing events to more than 70 teams
of conventional astronomers, who will use their optical, radio
and space-based telescopes to see whether those events produced
any form of electromagnetic radiation.
In return, the LIGO and
Virgo collaborations will be sifting through data to search for
gravitational waves that could have been generated by events,
such as supernova explosions, seen by the conventional
observatories.
Some 20 teams tried to follow up on
'the Event', mostly to no avail.
NASA's Fermi Gamma-ray Space
Telescope did see a possible burst of γ-rays about 0.4 seconds
later, coming from an equally vague but compatible region of the
southern sky.
4
But most observers now consider it to be a coincidence.
Such
γ-rays could, in principle, have been produced when gas orbiting
the binary black hole was heated up during the merger, says Vicky Kalogera, a LIGO astrophysicist at Northwestern University
in Evanston, Illinois.
But,
"our astrophysical expectation has
been that the gas from stars that formed the binary black hole
has long dispersed. There shouldn't be any significant gas
around", she says.
Going forward, however, matching
gravitational waves with electromagnetic ones could
usher in a new era of astronomy. In particular, mergers of
neutron stars are expected to produce short γ-ray bursts.
Researchers could then measure how far the light from those
bursts is shifted towards the red end of the spectrum, which
would tell astronomers how fast the stars' host galaxies are
receding owing to the expansion of the Universe.
Matching those
redshifts to distance
measurements calculated from gravitational waves should give
estimates of the current rate of cosmic expansion, known as the
Hubble constant, that are independent - and potentially more
precise - than calculations using current methods.
"From the point of view of
measuring the Hubble constant, that's our gold-plated
source", says Holz.
The LIGO and Virgo teams estimate
that they have a 90% chance of finding more events in the data
that LIGO has already collected.
They are confident that by the
time the next run finishes, the event count will be at least 5,
growing to perhaps 35 by the end of a run scheduled to start in
2017.
"To be honest," says Holz, "I
find it really hard to believe that the Universe is really
doing this stuff. But it's not science fiction. It really
happened."
References
-
Abbott, B. P. et al. Phys. Rev.
Lett. 116, 061102 (2016) -
Observation of Gravitational Waves
from a Binary Black Hole Merger
-
Abbott, B. P. et al. Astrophys.
J. Lett. 818, L22 (2016) -
Astrophysical Implications of The
Binary Black Hole Merger GW150914
-
Portegies Zwart, S. F.,
Baumgardt, H., Hut, P., Makino, J. & McMillan, S. L. W.
Nature 428, 724–726 (2004) -
Formation of massive black holes
through runaway collisions in dense young star clusters
-
Connaughton, V. et al. Preprint
at
Fermi GBM Observations of LIGO
Gravitational Wave event GW150914 (2016)
|