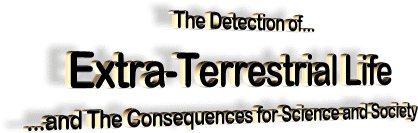
by Martin Dominik and John C. Zarnecki
25-26 January 2010
from
TheRoyalSociety Website
Authors Affiliations
M. Dominik - SUPA, University
of St Andrews, School of Physics and Astronomy, North Haugh, St
Andrews KY16 9SS, UK
J.C. Zarnecki - Planetary and Space Sciences Research Institute (PSSRI),
The Open University, Walton Hall, Milton Keynes MK7 6AA, UK
*Author for correspondence (md35@st-andrews.ac.uk).
PDF format |
Abstract
Astronomers are now able to detect planets orbiting stars other than
the Sun where life may exist, and living generations could see the
signatures of extra-terrestrial life being detected.
Should it turn
out that we are not alone in the Universe, it will fundamentally
affect how humanity understands itself - and we need to be prepared
for the consequences.
A Discussion Meeting held at the Royal Society
in London, 6-9 Carlton House Terrace, on 25-26 January 2010,
addressed not only the scientific but also the societal agenda, with
presentations covering a large diversity of topics.
1. The quest for
exploration
A thin layer around the surface of Earth is teeming with life of
huge diversity: from micro-organisms to plants and animals, and even
intelligent species.
Up to now, this forms the only known sample of
life in the Universe. However, observing the pinpoints of light on
the night sky has probably always inspired humans to speculate about
the existence of other worlds. It is, therefore, not surprising that
there is a long history of thoughts about such a proposition (e.g.
[1-5]).
Despite the fact that it is straightforward to imagine that
stars other than the Sun would also host planets, speculations
turned into evidence only fairly recently: in 1992, the first planet
around a special type of stellar remnant, namely pulsars, was found
[6], and in 1995, the first detection of a planet around a star of
similar composition to the Sun, namely 51 Peg b, was reported [7].
The enormous progress in this field is reflected by the fact that,
as of 1 June 2010, more than 450 extra-solar planets1 are known.
While most of these are gas giants like Jupiter and Saturn, some
spectacular discoveries of about 20 planets of less than 10 Earth
masses (e.g. [8-13]) have already indicated that rocky planets with
conditions considered suitable to harbour life are probably rather
common. The discovery of a true sibling of our home planet,
therefore, seems to remain only a question of time (cf. [14,15]).
The active quest for extra-solar planets has opened a new chapter in
the book of the search for extra-terrestrial life. This was already
an active field of science with the exploration of the Solar System
by means of space probes, which gave rise to a ‘space age’ from 1957
when Sputnik-1, the first Earth-orbiting artificial satellite, was
launched.
Current technology allows us to land a robotic chemistry
laboratory on other Solar System bodies, or return samples to Earth,
the latter coming with the advantage of being able to adapt analysis
strategies to unexpected findings.
Based on our current
understanding,
Mars,
Europa,
Enceladus and, if we consider life
based on a liquid other than water,
Titan are the most promising
places for finding life signatures (cf. [16]).
A direct search for
life on Mars, rather than searching for evidence from fossils, was
carried out as early as 1976 with the two Viking landers. However,
the outcome of these experiments is still subject to an unresolved
controversy (cf. [16,17]).
A further opportunity to find alien life
forms is given by the study of meteorites found on Earth (cf. [17]),
where it is now well established that some of them originate from
Mars [18]. However, the exchange of biological material between
Solar System bodies might also mean that such life is not distinct
from ours, but rather shares a common origin.
Only shortly after the advent of the space age, it was proposed to
use radio telescopes to search for signals arising from
extra-terrestrial civilizations [19], while independently
preparations for such an experiment, ‘Project Ozma’, were already
under way [20].
This marked the birth of a scientific venture known
as the ‘Search for Extra-Terrestrial Intelligence’ or ‘SETI’ for
short (e.g. [21]).
Exploration of the unknown, making use of previously unavailable
technology, led to ‘ages of wonder’ [22], where prevailing concepts
have been challenged and new ideas and insight emerged.
The study of
the origins, evolution, distribution and future of life in the
Universe, for which the term ‘astrobiology’ has been coined
(following up on the earlier used ‘exobiology’), plays a critical
role in a continuing era of enlightenment.
2. Universality or
uniqueness?
We readily accept that the concepts of physics and chemistry apply
throughout the cosmos and are valid for all time, but should this
not make us wonder whether biology is universal as well [22], and
not just a special feature that only applies to planet Earth?
There is actually no lack of the building blocks of life; the number
of molecules fundamental to Earth’s biochemistry that have already
been found in the interstellar medium, planetary atmospheres and on
the surfaces of comets, asteroids, meteorites and interplanetary
dust particles is surprisingly rather large.
Giant ‘factories’,
where complex molecules are being synthesized, appear to make
carbonaceous compounds ubiquitous in the Universe (cf. [23]).
We are however left with a fundamental gap in understanding just at
the point where molecules become ‘alive’. Nevertheless, it has been
conjectured that life resembling that on Earth in its biochemistry
is a cosmic imperative [24,25], following from the deterministic and
reproducible nature of chemistry under given environment conditions,
and the reproducibility of optimization by selection [26] from a
large number of variants.
The latter is strongly supported by the
observed evolutionary convergence in the biological history on
Earth, but it cannot be ruled out with certainty that our existence
is a fluke arising from a highly improbable chance event (cf. [27]).
A strong case for the genesis of life being a ‘cosmic imperative’
would arise from the detection of a ‘shadow biosphere’ on Earth with
a distinct ‘tree of life’ [28-30].
So if there are alien civilizations at a comparable stage of
evolution, one might expect that they do not differ that much from
our own (cf. [27]).
However, with the Sun just about half-way
through its lifetime as a main-sequence star, with about 4.5 billion
years remaining, that ‘comparable stage’ might constitute a rather
short transient episode, and advanced extra-terrestrial life might
be inconceivable to us in its complexity, just as human life is to
amoebae.
3. Our lack of
knowledge and the arising challenges
The current state of the study of life in the Universe sees us being
confronted with many questions cutting across various traditional
fields of science, while leaving us with almost no answers.
The
inherent interdisciplinarity does not come as a surprise when
realizing that we are investigating ourselves,2 our origins and
future, and our role in the cosmos.
Our ignorance is most famously quantified by the
Drake equation
[30-32] which describes the number of civilizations N
that are detectable by means of electromagnetic emissions (more
particularly, radio signals) as a product of various factors, namely
the rate Graphic of formation of suitable stars, the fraction fp of
those with planetary systems, the number ne of planets per such
system with conditions suitable for life, the fraction fl of such
planets on which life actually develops, the fraction fi of
life-bearing planets on which intelligent life emerges, the fraction
fc of emerged civilizations that develop technologies for
propagating detectable signals and finally the time span L over
which these civilizations disseminate such signals.
Rather than as a
product of numbers, the Drake equation should more appropriately be
seen as a product of random variables with their respective
distribution functions [33-35].
Interestingly, the uncertainty among
the different factors in the Drake equation increases from left to
right. The ‘astronomical factors’ Graphic, fp and ne are rather well
determined as compared with the ‘biological factors’ fl and fi,
while the ‘technological factor’ fc and even more the ‘societal
factor’ L are the great unknowns.
Despite the fact that the Drake
equation has been devised for SETI, only the last three factors are
specific to intelligent life or its detection by means of
electromagnetic signals, whereas the others are relevant to any
astrobiological context.
Let us suppose that life beyond Earth does exist. In order to detect
it, we encounter substantial difficulties when aiming to define its
characteristics, and in selecting signatures that are certainly
incompatible with an abiogenic origin.
Organic molecules with a
carbon skeleton that are stable on geological time-scales form
‘chemical fossils’ that constitute an early record of life on Earth.
Moreover, measured carbon isotope ratios in sedimentary rocks
suggest the presence of microbial life already 3.8 billion years ago
([36]; cf. [17]). It, however, requires biological material to
determine whether life is truly ‘alien’, i.e. belonging to a ‘tree
of life’ distinct from that of life on Earth.
Evolutionary selection
is likely to result in the use of a set of basic organic molecules,
but it is a subject of debate whether there is a strong evolutionary
convergence either to the one and only optimum or in such a way that
the process of natural selection always leads to the same global
optimum for all environments under which life can evolve, or whether
a weak evolutionary convergence accounts for the possibility of
ending up with different optima for the realization of life or its
features.
Strikingly, a system of life based on molecules just of
opposite
chirality but otherwise identical to those that form the
building blocks for life known on Earth appears to be a viable
distinctive alternative (cf. [16,17,27]).
Out of the vast number of places in the Universe to look for life,
what should guide our search?
With no other account for life other
than that on Earth and a lack of understanding of the properties and
preferred environments of life as we do not know it, one readily
tends to accept the null hypothesis that an efficient search should
be oriented towards the set of conditions that is defined by the
variety of terrestrial life forms.
Therefore, a widely adopted
strategy is to search for liquid-water habitats, given that
terrestrial biochemistry relies on liquid water as solvent (cf. [16,27]).
Moreover, given the requirements of metabolism, energy is
a more universal imperative for life, providing a further criterion
to narrow down searches, and opening an opportunity to go far beyond
characteristics that might be specific to life as we know it [37].
While it was the porphyrin nucleus, central to the structure of
chlorophyll, that paved the way for using chemical fossils as
biomarkers ([38]; cf. [17]), even before the age of photosynthesis
life may have been living on energy sources bound within rocks, such
as iron.
Rather than just the presence of water or energy, it is the
kinetics of water flows that constitute the crucial criterion for
such processes to succeed (cf. [39]).
Not only has Earth initially provided an environment for life to
develop, but also the resulting living organisms have subsequently
shaped the planet. In particular, the large abundance of oxygen in
the Earth’s atmosphere is the result of biogenic photosynthesis.
Such feedback mechanisms gave rise to the idea of describing the
Earth’s biosphere, atmosphere, oceans and soil as a complex entity
in what is referred to as the ‘Gaia theory’ [40,41].
In fact, it
emerged from thoughts about simple signatures of life on another
planet [42], and given that planets outside the Solar System cannot
be explored by spacecraft, measurements of the abundance of
molecules in the planetary atmosphere from related spectral features
in order to construct a biosignature are the very limited ‘bits and
pieces’ of information upon which we can draw conclusions about
life.
Such efforts mark one of the greatest challenges ever
undertaken in observational astronomy (cf. [15]).
4. Societal
relevance and political action
The detection and further study of extra-terrestrial life will
fundamentally challenge our view of nature, including ourselves, and
therefore the field of astrobiology can hardly be isolated from its
societal context, including philosophical, ethical and theological
perspectives (cf. [43]).
With the detection of extra-terrestrial life being technically
feasible, one needs to address whether perceived societal benefits
command us to search for it, or whether such an endeavor may rather
turn out to be a threat to our own existence (cf. [44]).
Modeled
after
the Torino Scale for asteroid/comet impact predictions [45]
and the Rio Scale for a putative discovery of extra-terrestrial
intelligence [46], the London Scale index (LSI) with values ranging
from 0 to 10 together with an independently evaluated level of risk
or biohazard [47] provides an assessment of the scientific
importance, validity and potential risks associated with putative
evidence of extra-terrestrial life discovered on Earth, on nearby
bodies in the Solar System, or in our Galaxy.
Various scenarios of encounters with extra-terrestrial life have
already been portrayed in the science-fiction literature and films,
some of these being more scientific, others more fictional (cf.
[48]).
Imagination, however, must not be underestimated as a
valuable means to advance knowledge towards new frontiers, and is
not at all an unscientific concept. It is also valuable that a broad
public has been given the opportunity to reflect on this topic.
Similarly, scientists involved in relevant research themselves
should engage with journalists and the public (cf. [49]).
Media
reports and weblogs debating extra-terrestrial life, including those
that relate to this very Royal Society Discussion Meeting, also
provide some evidence on public opinion and reactions that can be
expected.
If data are absent or ambiguous, we tend to argue by retreating to
analogies or theories about universalities. Historical examples,
however, need to be well understood before these can serve as a
guide, which is demonstrated by the fact that history is full of
misinterpretations and misconceptions of itself (cf. [48,49]).
Rather than aliens invading Earth, most likely detection scenarios
will involve microbial organisms and/or extra-terrestrial life at a
safe distance that prevents physical contact. As far as exploring
other life forms is concerned, any strategy applied must exclude
biological contamination - not only to protect ourselves, but also to
preserve any alien life discovered as part of an overall commitment
to enhancing the richness and the diversity of life in the Universe
[16].
For such scenarios with well-contained risks, the dominant
human response is unlikely to be one of fear and pandemonium [48].
Human perceptions and representations of alien life will not only
derive from science, but, given that humanity is more than just a
collection of logic and facts, they will be highly influenced by
cultural and psychological factors. Therefore, reactions will not
necessarily be homogeneous, and reality may defy common myths [49].
It is believed by some that establishing the presence of
extra-terrestrial life as a fact will cause a crisis for certain
religious faiths. A survey, however, shows that followers of all the
main religious denominations as well as atheists declare that it
will not be a problem for their own beliefs [50].
While scientists are obliged to assess benefits and risks that
relate to their research, the political responsibility for decisions
arising following the detection of extra-terrestrial life cannot and
should not rest with them. Any such decision will require a broad
societal dialogue and a proper political mandate. If
extra-terrestrial life happens to be detected, a coordinated
response that takes into account all the related sensitivities
should already be in place.
In 1989, the International Academy of
Astronautics (IAA) approved a SETI post-detection protocol [51],
which was developed by one of its committees.
Despite the fact that
it has subsequently been endorsed by,
-
the International Institute of
Space Law (IISL)
-
the Committee on Space Research (COSPAR) of the
International Council for Science (ICSU)
-
the International
Astronomical Union (IAU)
-
the International Union of Radio
Science (URSI),
...the procedures laid out in that document are not
legally enforceable.
If it remains a voluntary code of practice, it
will probably be ignored in the event to which it should apply.
Will
a suitable process based on expert advice from proper and
responsible scientists arise at all, or will interests of power and
opportunism more probably set the scene (cf. [52])?
A lack of
coordination can be avoided by creating an overarching framework in
a truly global effort governed by an international politically
legitimated body. The United Nations fora constitute a ready-made
mechanism for coordination.
Member States of the Committee on the
Peaceful Uses of Outer Space (COPUOS) will need to place
‘supra-Earth affairs’ on the agenda in order to take it further to
the General Assembly, with the goal of establishing structures
similar to those created for dealing with threats arising from
potentially impacting near-Earth objects [53].
5. Outlook
So far, there is no scientific evidence for or against the existence
of life beyond Earth.
All arguments about whether life is common and
universal or whether we live in a unique place in the cosmos are
rather based on philosophical beliefs and assumptions. Consequently,
there is no way of predicting the outcomes of searches for
extra-terrestrial life. This, however, surely drives the scientific
imperative to test the hypothesis.
The year 2010 marks the 50th anniversary of the first search for
radio signals originating from other civilizations, a remarkably
optimistic endeavor in 1960, particularly bearing in mind that up
to now all SETI experiments have provided a negative result.
One,
however, has to realize that these have probed only our neighborhood, up to about 200 light-years distant, whereas the
centre of the Milky Way is 25,000 light-years away from us. And even
if there is no other intelligent life in the Milky Way, it could
still be hosted in another of the remaining hundreds of billions of
other galaxies.
Advanced efforts are now on the drawing board or already under way
for the further exploration of the Solar System and the search for
biomarkers in the atmospheres of extra-solar planets, while searches
for signals of extra-terrestrial intelligence are entering a new era
with the deployment of the next generation of radio telescopes.
The study and understanding of life in the Universe encompasses
many, if not all, of the fundamental questions in biology, physics
and chemistry, but also in philosophy, psychology, religion and the
way in which humans interact with their environment and each other.
While we cannot be prepared for the unpredictable, the careful
development of a societal agenda alongside a scientific agenda for
the search for life elsewhere becomes mandatory.
Frequently, things are only seen in the proper context if observed
from a far enough distance. The image of Earth taken by Voyager 1
from as near as about 40 AU, i.e. still within the outer regions of
the Solar System, which depicts just a ‘pale blue dot’, proves
insightful.
As
Carl Sagan [54] (p. 9) worded it:
‘Our posturings,
our imagined self-importance, the delusion that we have some
privileged position in the Universe, are challenged by this point of
pale light.’
For the first time in human history, living generations are now
given a realistic chance to find out whether we are alone in the
Universe.
Should an answer be found one day, we will still be left
with deeper questions to be answered: where do we come from, why are
we here and where will we be going?
Acknowledgements
We would like to express our thanks to the session chairs of the
meeting, namely Steven Rose, Catherine Cesarsky, Jocelyn Bell-Burnell
and Martin Rees, the reviewers of the papers in this issue, Uffe G.
Jørgensen for a careful reading of this manuscript, Paul Browne and
Christine Liebig for handling the microphones, and finally the event
managers of the Royal Society, as well as the IT, catering and other
staff without whom the meeting would not have been as enjoyable as
it was.
Footnotes
One contribution of 17 to a Discussion Meeting Issue ‘The detection
of extra-terrestrial life and the consequences for science and
society’.
1 - This number is likely to be out of date already by the time this
paper is published, but the reader is referred to the ‘Extrasolar
Planets Encyclopedia’ at http://exoplanet.eu.
2 - As Frank Drake likes to point out.
References
-
Dick S. J. - (1982) Plurality of
worlds: the origins of the extraterrestrial life debate from
Democritus to Kant (Cambridge University Press, Cambridge,
UK).
-
Dick S. J. - (1996) The
biological universe: the twentieth century extraterrestrial
life debate and the limits of science (Cambridge University
Press, Cambridge, UK).
-
Dick S. J. - (2001) Life on
other worlds: the 20th-century extraterrestrial life debate
(Cambridge University Press, Cambridge, UK). Crowe M. J.
- (1986) The extraterrestrial life debate, 1750-1900
(Cambridge University Press, Cambridge, UK).
-
Crowe M. J. - (2008) The
extraterrestrial life debate, antiquity to 1915: a source
book (University of Notre Dame Press, Notre Dame, Indiana).
-
Wolszczan A., Frail D. A. - 1992
A planetary system around the millisecond pulsar PSR1257+12
Nature 355 145 147 (doi:10.1038/355145a0)
-
Mayor M., Queloz D. - 1995 A
Jupiter-mass companion to a solar-type star Nature 378 355
359 (doi:10.1038/378355a0)
-
Rivera E. J., Lissauer J. J.,
Butler R. P., Marcy G. W., Vogt S. S., Fischer D. A., Brown
T. M., Laughlin G., Henry G. W. - 2005 A - 7.5 M- planet
orbiting the nearby star, GJ 876 Astrophys. J. 634 625 640
(doi:10.1086/491669)
-
Beaulieu J.-P., et al. - 2006
Discovery of a cool planet of 5.5 Earth masses through
gravitational microlensing Nature 439 437 440
(doi:10.1038/nature04441)
-
Udry S., et al. - 2007 The HARPS
search for southern extra-solar planets. XI. Super-Earths (5
and 8 M⊕) in a 3-planet system Astron. Astrophys. 469 L43
L47 (doi:10.1051/0004-6361:20077612)
-
Mayor M., - et al. - 2009 The
HARPS search for southern extra-solar planets. XVIII. An
Earth-mass planet in the GJ 581 planetary system Astron.
Astrophys. 507 487 494 (doi:10.1051/0004-6361/200912172)
-
Léger A., et al. - 2009
Transiting exoplanets from the CoRoT space mission. VIII.
CoRoT-7b: the first super-Earth with measured radius Astron.
Astrophys. 506 287 302 (doi:10.1051/0004-6361/200911933)
-
Queloz D., et al. - 2009 The
CoRoT-7 planetary system: two orbiting super-Earths Astron.
Astrophys. 506 303 319 (doi:10.1051/0004-6361/200913096)
-
Mayor M., Udry S., Pepe F.,
Lovis C. - 2011 Exoplanets: the quest for Earths twin Phil.
Trans. R. Soc. A 369 572 581 (doi:10.1098/rsta.2010.0245)
-
Fridlund M. - 2011
Extra-terrestrial life in the European Space Agency’s Cosmic
Vision plan and beyond Phil. Trans. R. Soc. A 369 582 593
(doi:10.1098/rsta.2010.0233)
-
McKay C. P. - 2011 The search
for life in our Solar System and the implications for
science and society Phil. Trans. R. Soc. A 369 594 606
(doi:10.1098/rsta.2010.0247)
-
Pillinger C. - 2011 Chemical
methods for searching for evidence of extra-terrestrial life
Phil. Trans. R. Soc. A 369 607 619
(doi:10.1098/rsta.2010.0241)
-
McSween H. J. - 1984 SNC
meteorites: are they Martian rocks? Geology 12 3 6
(doi:10.1130/0091-7613(1984)12<3:SMATMR>2.0.CO;2)
-
Cocconi G., Morrison P. - 1959
Searching for interstellar communications Nature 184 844 846
(doi:10.1038/184844a0)
-
Drake F. D. - 1961 Project Ozma
Phys. Today 14 40 46 (doi:10.1063/1.3057500)
-
Tarter J. - 2001 The search for
extraterrestrial intelligence (SETI) Annu. Rev. Astron.
Astrophys. 39 511 548 (doi:10.1146/annurev.astro.39.1.511)
-
Blumberg B. S. - 2011
Astrobiology, space and the future age of discovery Phil.
Trans. R. Soc. A 369 508 515 (doi:10.1098/rsta.2010.0239)
-
Ehrenfreund P., Spaans M., Holm
N. G. - 2011 The evolution of organic matter in space Phil.
Trans. R. Soc. A 369 538 554 (doi:10.1098/rsta.2010.0231)
-
de Duve C. - (1995) Vital dust:
life as a cosmic imperative (Basic Books, New York, NY).
-
de Duve C. - 2011 Life as a
cosmic imperative? Phil. Trans. R. Soc. A 369 620 623
(doi:10.1098/rsta.2010.0312)
-
Darwin C. R. - (1859) On the
origin of species by means of natural selection, or the
preservation of favoured races in the struggle for life
(John Murray, London, UK).
-
Conway Morris S. - 2011
Predicting what extra-terrestrials will be like: and
preparing for the worst Phil. Trans. R. Soc. A 369 555 571
(doi:10.1098/rsta.2010.0276)
-
Cleland C. E., Copley D. C. -
2005 The possibility of alternative microbial life on Earth
Int. J. Astrobiol. 4 165 173 (doi:10.1017/S147355040500279X)
-
Davies P. C. W., Lineweaver C.
H. - 2005 Finding a second sample of life on Earth
Astrobiology 5 154 163 (doi:10.1089/ast.2005.5.154)
-
Davies P. C. W. - 2011 Searching
for a shadow biosphere on Earth as a test of the ‘cosmic
imperative’ Phil. Trans. R. Soc. A 369 624 632
(doi:10.1098/rsta.2010.0235)
-
Drake F. - 2011 The search for
extra-terrestrial intelligence Phil. Trans. R. Soc. A 369
633 643 (doi:10.1098/rsta.2010.0282)
-
Drake F. D. - (1962) Intelligent
life in space (Macmillan, New York, NY).
-
Maccone C. - (2008) The
statistical Drake equation. Paper no. IAC-08-A4.1.4,
presented at the 59th Int. Astronautical Congress (IAC) in
Glasgow, UK, 29 September to 3 October 2008.
-
Maccone C. - In press The living
Drake equation of the Tau Zero Foundation Acta Astron.
(doi:10.1016/j.actaastro.2010.03.016)
-
Forgan D. H. - 2009 A numerical
testbed for hypotheses of extraterrestrial life and
intelligence Int. J. Astrobiol. 8 121 131
(doi:10.1017/S1473550408004321)
-
Schidlowski M. - 1988 A
3800-million-year isotopic record of life from carbon in
sedimentary rocks Nature 333 313 318 (doi:10.1038/333313a0)
-
Hoehler T. M., Amend J. P.,
Shock E. L. - 2007 ‘follow the energy’ approach for
astrobiology Astrobiology 6 819 823
(doi:10.1089/ast.2007.0207)
-
Treibs A. - 1934 Über das
Vorkommen von Chlorophyllderivaten in einem Ölschiefer aus
der oberen Trias Justus Liebigs Ann. Chem. 509 103 114
(doi:10.1002/jlac.19345090108)
-
Cockell C. S. - 2011 Life in the
lithosphere, kinetics and the prospects for life elsewhere
Phil. Trans. R. Soc. A 369 516 537
(doi:10.1098/rsta.2010.0232)
-
Lovelock J. E. - 1972 Gaia as
seen through the atmosphere Atmos. Environ. 6 579 580
(doi:10.1016/0004-6981(72)90076-5)
-
Lovelock J. E. - (1979) Gaia: a
new look at life on Earth (Oxford University Press, Oxford,
UK).
-
Lovelock J. E. - 1965 A physical
basis for life detection experiments Nature 207 568 570
(doi:10.1038/207568a0)
-
Bertka C. M. - ed (2009)
Exploring the origin, extent, and future of life -
philosophical, ethical and theological perspectives
(Cambridge University Press, Cambridge, UK).
-
Michaud M. A. G. - (2006)
Contact with alien civilizations - our hopes and fears about
encountering extraterrestrials (Springer, Berlin, Germany).
-
Binzel R. P. - 1997 A near-Earth
object hazard index Ann. NY Acad. Sci. 822 545 551
(doi:10.1111/j.1749-6632.1997.tb48366.x)
-
Almár I., Tarter J. - 2011 The
discovery of ETI as a high-consequence, low-probability
event Acta Astron. 68 358 361
-
doi:10.1016/j.actaastro.2009.07.007)
-
Almár I., Race M. S. - 2011
Discovery of extra-terrestrial life: assessment by scales of
its importance and associated risks Phil. Trans. R. Soc. A
369 679 692 (doi:10.1098/rsta.2010.0227)
-
Harrison A. A. - 2011 Fear,
pandemonium, equanimity and delight: human responses to
extra-terrestrial life Phil. Trans. R. Soc. A 369 656 668
(doi:10.1098/rsta.2010.0229)
-
Denning K. - 2011 Is life what
we make of it? Phil. Trans. R. Soc. A 369 669 678
(doi:10.1098/rsta.2010.0230)
-
Peters T. - 2011 The
implications of the discovery of extra-terrestrial life for
religion Phil. Trans. R. Soc. A 369 644 655
(doi:10.1098/rsta.2010.0234)
-
Tarter J., Michaud M. A. - eds
(1990) SETI post-detection protocol. Acta Astronaut.
71:(special issue).
-
Sagan C. - (1985) Contact (Simon
& Schuster, New York, NY).
-
Othman M. - 2011 Supra-Earth
affairs Phil. Trans. R. Soc. A 369 693 699
(doi:10.1098/rsta.2010.0311)
-
Sagan C. - (1994) Pale blue dot:
a vision of the human future in space (Random House, New
York, NY).
|