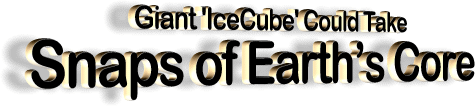
by Stephen Battersby
New Scientist News Service
23 November 2007
from
SpaceNewScientist Website
A giant telescope buried in ice at the
South Pole could one day create pictures of the Earth’s core.
According to a new calculation, the instrument – called IceCube
– could produce a picture of the Earth's dense iron core,
silhouetted against the lighter rocky mantle.
Currently under construction, IceCube is designed to detect
subatomic particles called neutrinos, which are so evasive that they
can slip quite easily through the body of the planet.
A few neutrinos are not so lucky, however, and deep under the
South Pole IceCube is designed to spot them. The machine
consists of thousands of detectors and will eventually fill a cubic
kilometer of ice. The detectors look downwards, watching for the
distinctive flash of blue light that means a neutrino has come
through most of the planet only to get snagged in the Antarctic ice.
The main aim is to look for neutrinos from exotic objects in deep
space, such as the giant black holes in galactic cores, using the
bulk of the Earth as a shield to screen out unwanted noise from
other cosmic particles.
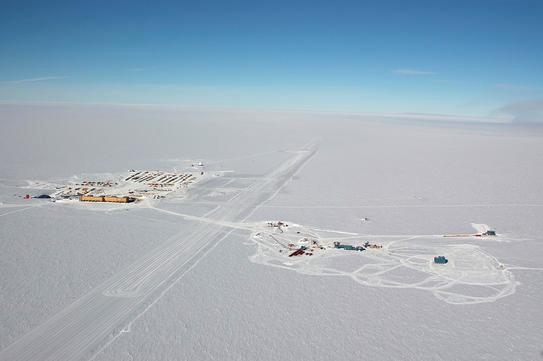
An overview of the
South Pole with the South Pole Station to the left of the runway
and the IceCube
construction site to the right.
(Image: Forest
Banks/NSF)
Scanning Earth
But IceCube could also
serve as a planetary scanner. Some neutrinos have exceedingly high
energy, above 10 trillion electronvolts (millions of times more than
the typical energies of nuclear radiation). These energetic
particles are not quite so slippery, and a large fraction of them
should be stopped by the Earth's layers of iron and rock.
Astronomers had hoped to find a powerful beam of neutrinos from a
distant source – perhaps one of those giant black holes – which they
could then monitor with IceCube as the Earth rotates, scanning the
beam's path across the planet to reveal its structure.
Unfortunately IceCube's predecessor, a neutrino telescope called
AMANDA, can't see any of these distant neutrino sources.
They must all be fairly faint.
"We will see them with IceCube, but
they are not intense enough to scan the Earth," says Maria
Gonzalez-Garcia of Barcelona University.
Instead, Gonzalez-Garcia and her
colleague have worked out that IceCube can use a diffuse glow of
neutrinos created in Earth's upper atmosphere by the impact of
energetic protons and other cosmic rays.
Sharper vision
The dense iron at Earth's core should
absorb more of these atmospheric neutrinos than the rock of the
surrounding mantle. So with Earth illuminated from behind, IceCube
should be able to see the core standing out as a dark shadow – just
like a bone revealed by X-rays.
Another large neutrino telescope called KM3NeT, planned for the deep
clear waters of the Mediterranean, could give us a second opinion.
In principle, this method could have sharper vision than at least
one of the conventional methods for probing the Earth's interior, in
which scientists calculate the core's shape by measuring the way the
planet wobbles.
However, the neutrino illumination is so dim that a picture can only
be built up very slowly. After a decade-long exposure with IceCube,
the authors calculate that there should be an outline of the core,
but seeing any detail at the core-mantle boundary would take much
longer – or better, a still vaster neutrino telescope.
"With a bigger detector you can be
more precise," Gonzalez-Garcia told New Scientist.
Caltech Earth scientist David
Stevenson points out that much more detailed pictures can
already be built up by analyzing seismic waves travelling through
the planet.
"I am a proponent of neutrino
geophysics, but it will probably not have a major payoff for
decades."
Telescope to Be Under South Pole - Buried
'Icecube': Neutrino Detector Will Use Earth's Diameter as Shield
Unique Buried South Pole
Telescope Points To Earth's Center
Original headline: LONDON
from
FarShores Website
You don't have to be the Astronomer
Royal to understand a few basic rules about building telescopes:
They should have a good view of the sky, they should be easily
accessible and it's probably best to point them upwards.
Think again. Astronomers are completing plans for a telescope buried
under the South Pole. One cubic kilometer large, it will point down
through the centre of the Earth toward the northern skies above
Europe, Asia, North America and the Arctic.
This is the IceCube, the strangest, most isolated and
certainly coldest terrestrial telescope ever planned. The IceCube is
a neutrino detector created to seek out ghostly, high-energy
sub-atomic particles from the farthest reaches of space. It will be
made by drilling 2.5-kilometre-deep holes in the ice with hot water,
then dropping in strings of light detectors assembled like pearls on
a thread.
These plans were given a boost this month with the publication in
Nature of the first results from the IceCube's sister, Amanda,
or the Antarctic Muon and Neutrino Detector Array.
Those results are being discussed this week at the U.K. National
Astronomical Meeting in Cambridge.
Neutrinos are the most elusive particles known. They have almost no
mass, no charge and can flit through planets - or the centre of
galaxies - at the speed of light. They travel the largest imaginable
distances unabsorbed by matter or radiation and so are unhindered by
stars, planets or magnetic fields.
Most neutrinos detected on Earth come from the atmosphere, where
they are generated by cosmic rays hitting air molecules, or from the
sun. But high-energy neutrinos are also produced in collisions in
some of the most violent places in the universe.
By detecting the particles and following their trails back to their
point of origin, physicists hope to get unparalleled insight into
colliding black holes, gamma-ray bursts -- the mysterious explosions
that light up the cosmos once a day -- the wreckage of exploded
stars and the violent cores of distant galaxies.
To investigate neutrinos, physicists need a dense shield that
filters out other less persistent particles. In a laboratory, that
is usually made from lead. But when it comes to looking at cosmic
neutrinos, a far bigger and more sensitive detector is needed. One
solution is to use Earth itself as a shield and adapt the properties
of the clear ice of the South Pole.
The principle has been shown to work with Amanda, built in the 1990s
at the U.S. Amundsen-Scott South Pole Station. It is designed
to look down to the sky in the Northern hemisphere using the Earth
to block out everything else.
Francis Halzen, a physicist at the University of
Wisconsin-Madison who led the study, says:
"We have proved the technique. We
have a unique probe with a sensitivity well beyond other
experiments, and the neutrinos we've seen are of a higher energy
than has been seen before."
Amanda is made from 677 glass
modules, each the size of a bowling ball, strung out on electrical
cables beneath the ice and arranged in a cylinder 500 meters tall
and 120 meters in diameter. The array detects the one in 10 billion
neutrinos travelling the distance of Earth's diameter that happens
to collide with a proton.
The collision produces another type of sub-atomic particle known as
a
muon. These particles travel close
to the speed of light in a vacuum but exceed the speed of light in
water or ice. The result is Cherenkov radiation, the optical
equivalent of a sonic boom. The muon leaves behind a
trail of blue light identical to the path of the neutrino. Amanda's
modules, or photomultiplier tubes, work like light bulbs in reverse
by capturing these faint streaks and sending information about their
direction and energy levels to the surface.
The streaks can also be detected in water and have been picked up by
a smaller telescope in the clear seas of Hawaii. Proposals have been
made to create another detector in the Mediterranean that will
measure neutrinos coming in from the southern sky. Ice scatters
light more, but absorbs it less. The ice detectors will gauge energy
levels more accurately, while deep-sea detectors will be better at
tracking direction.
The team has a sample of a few hundred neutrinos detected by Amanda.
"All other properties of these
neutrinos agree with their atmospheric origin," says Halzen. "We
actually show that no more than 15% of the sample could possibly
have a different origin. At present, we are looking for cosmic
neutrinos, for instance those from gamma-ray bursts and active
galaxies. The IceCube will be built around Amanda. The
$65-million array will be made from 4,800 optical sensors on 80
strings. Each string will be 125 meters apart and have 60
modules spaced at 16m intervals.
"It is not only bigger; it is a much
better instrument than Amanda," says Halzen. "It can identify
neutrino flavours, or types, and has far superior resolution."
The uppermost modules will be more than
1 kilometer beneath the surface.
The array should be big enough to pick up astronomical neutrinos
moving up through the ice. Those moving down will be mostly muons
produced by cosmic rays in the atmosphere. The array will also be
able to reveal the neutrino type, or flavor.
The hope is that the IceCube will answer questions about gamma-ray
bursts, as well as about neutrinos. Two years ago, a team of 100
Japanese and American physicists studying neutrinos in a vast tank
of water inside a mountain found compelling evidence that the
particles have mass.
They believe that though, individually, a neutrino's mass is
negligible, collectively they may account for a significant fraction
of the missing mass of the universe -- the substance known as "dark
matter."
The findings came from Super-Kamiokande, a detector made from a
60-million-litre cavity carved beneath mountains in Japan and lined
with steel. The tank contained 13,000 light detectors that could
spot Cherenkov radiation. The detector showed that muon
neutrinos vanished and reappeared as they passed through the Earth.
It seems unlikely that energy and momentum are really disappearing
from the universe. A more plausible explanation is that neutrinos
are changing as they pass through space into types that cannot be
detected. And this, the researchers concluded, was possible only if
the neutrino had mass.
The IceCube is still at the planning stage, but could be in place
within five or six years. If all goes well, Halzen and his
colleagues believe they will soon be embarking on a new era of
astronomy.
|