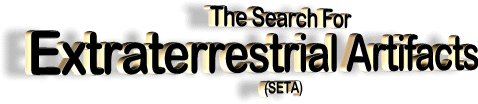
by Robert A. Freitas Jr.
Xenology Research Institute
8256 Scottsdale Drive, Sacramento,
California 95828, USA
from
SearchForExtraTerrestrialVisitation
Website
The Journal of the British
Interplanetary Society, Vol. 36, pp. 501-506, 1983
Permission for reproducing was granted by the original
author.
The Artifact Hypothesis
states that an advanced extraterrestrial intelligence
has undertaken a long-term programme of galactic
exploration via the transmission of material artifacts.
An attempt to verify this hypothesis experimentally, the
search for extraterrestrial artifacts (SETA), is
proposed to detect such evidence in the Solar System by
telescopic, radar, infrared, direct probe, or other
available means. |
1. THE ARTIFACT
HYPOTHESIS
Current scientific interest in the search for extraterrestrial
intelligence (SETI) is motivated by the recognition that the
technology for interstellar communication is now available [1]. In
recent decades many searches for interstellar radio beacons and
signals have been proposed and actually conducted [2-4].
The two principle assumptions in all
such efforts are that,
(1) advanced extraterrestrial
intelligence exists in the Universe
(2) these intelligences are
presently attempting to locate, examine, or possibly
communicate with us
A previous paper [5] argues that
interstellar spacecraft are generally preferable to electromagnetic
wave propagation for extrasolar exploration and communication.
Further, recent objections to the existence of extraterrestrial
intelligence based on the
Fermi Paradox [6-8] are invalid [9]
because they are based on the unsupported assumption that ETI or
their artifacts are not now present in the Solar System.
Our ignorance of potential evidence of
ETI in the Solar System is not generally appreciated. (As in Ref. 9,
it is conceded that the UFO controversy and terrestrial sightings
have no direct relevance to the present observational question). In
view of these results, and in order to subject the Fermi Paradox to
needed experimental testing, I offer the Artifact Hypothesis:
A technologically advanced
extraterrestrial civilization has undertaken a long-term
programme of’ interstellar exploration via transmission of
material artifacts.
If the hypothesis is correct, then
unless the programme has only just begun, some evidence of this
extraterrestrial exploratory activity should be apparent within the
confines of the Solar System and thus could be detected by a
suitable observational effort [10-11].
On the other hand, if testing
by observation disproves the Hypothesis, and if the arguments for
physical probe superiority are regarded as conclusive, then the case
for the nonexistence of ETI based on the Fermi Paradox becomes far
more compelling.
2. THEORETICAL
BACKGROUND
The nature of observable artifacts depends in part upon unknown
alien motives for sending them.
Artifacts not intended to be found
will not be found. For instance, in one scenario the probe
imperfectly camouflages itself with the motive of providing a thresholding test of the technology or intelligence of the recipient
species, which test must be passed before communication with the
device is permitted.
The technological superiority of the
sending civilization guarantees the efficacy of the mission design
and the impossibility of discovering the artifact until the
predetermined conditions for discovery are satisfied. Since these
conditions cannot be specified a priori, the result is an
observationally null set. In another plausible scenario, the probe
hides to ensure the military security of the sending civilization
during clandestine surveillance of the target star system. Again the
search space is observationally null because of the necessary
technological impenetrability of the disguise.
Thus only those classes of artifacts not subject to a policy of
perfect concealment can be observed by us. Observable evidence will
be provided by ETI who do not particularly care whether we find them
or not [12], or who may actually be interested in communicating with
us yet be unwilling or only conditionally willing to initiate
contact. This may imply careful and unobtrusive surveillance by ETI,
with no special effort to disguise the alien presence.
Artifact base sites would then be chosen
strictly for reasons of efficiency, maintainability, or low
environmental risk, and so should be observable by us. This is the
most conservative assumption from the standpoint of the Fermi
Paradox, exemplified by many pretechnological peoples on Earth who
even today have little knowledge of the modern world. The more
restrictive assumption that ETI activities are perfectly concealed
leads to a trivial resolution of the Fermi Paradox.
There are four classes of unconcealed,
potentially observable artifacts, as follows.
2. 1 Astroengineering
If a highly exploitative civilization exists or had ever existed
in our vicinity, then the Solar System would have been wholly
converted to replicating machine mass (“industri-forming”.[13] )
and the Sun stripped of its fuel. In this case the existence of
mankind is negative evidence ruling out such activity.
Stephenson [14] suggests that Pluto’s unusual orbit may be
evidence of past extraterrestrial tampering, and Papagiannis
[15] speculates that the Asteroid Belt may be a giant slag heap
left over from ETI heavy industry.
But the existence of Saturn’s rings,
eccentric comets, Uranus’ axial tilt, Triton’s retrograde orbit,
Venus’ backwards rotation, the
Titus-Bode rule for planetary
spacing, even the evolution, of life on Earth all could provide
similar “evidence.” Without corroboration, none is persuasive
because in each case more prosaic explanations exist.
Kuiper and Morris [12] and
Stephenson [16] argue that the only plausible interstellar
mission is one of pure exploration - the pursuit of knowledge as
a source of wealth - and Tipler [17] points out that this
information theory of wealth is widely held by modern
economists, all of which supports the iraplausibility of
large-scale rapacious activities by ETI.
2. 2 Self-Replicating Artifacts
Self-reproducing machine systems may be present in the Solar
System which are building and launching interstellar probes
bound for other star systems (exploration motive); organizing
local resources for out-shipment to materials-hungry or “energy
crisis” extraterrestrial civilizations (exploitation motive); or
helping interstellar arks [18, 19], space colonies [15], crewed
interstellar probes [14] or habitats [20-21] to refuel, rebuild,
repair, grow or replicate (depot motive) or to settle here
permanently (colonization motive).
Also, we might hope to observe the
environmental aftermath of such activities, including [22] piles
of rubble or debris, palcomagnetic anomalies, radioactive hot
spots, derelict machinery or tools, abandoned strip mining pits,
rocket plumes from departing replicated daughter probes, and so
forth. While possible in principle, none of the above is likely
to be observable in any reasonable time frame.
Self-replicating or self-growing
probe factories need only produce a dozen or fewer offspring in
each target star system to explore the entire Galaxy in less
than a dozen generations, requiring 102-103 years for completion
of one generation at each site [23-24].
This is 10-6 – 10-7 the age of the
Earth, an improbably small observational window. Individual
replicating systems may be 100 meters in diameter [24], so a
fully-grown/replicated factory system for building probes [23]
need not exceed 0.1-1 km in size.
This is roughly 10-12 of the total
surface area of all known bodies in the Solar System, so even if
the search can be limited by siting logic and many such devices
have arrived here and are still active they will be extremely
difficult to detect. Interplanetary non-replicating subprobes
built and launched (perhaps into Earth orbit) by the factory are
more likely to be observed than the factory itself.
However, a good case can be made that no exploratory replicating
systems will be sent to the Solar System at all. Unless life is
extremely widespread most star systems will be uninhabited.
Hence the argument that humanity’s value as an undisturbed
intelligence increases with the rarity of the sentience in the
Galaxy [16] implies ETI will erect their self-replicating probe
factories in obviously uninhabited star systems and just send
non-reproducing exploratory probes to the fewer more promising
systems.
This avoids disturbing valuable
indigenous intelligent species which may later come under
observation. Exploitative machinery has no reason to stop short
of astrophagy, which is not observed. Interstellar arks using
the Solar System as a supply depot would have an unobservably
short residence time before returning to their native
inter-stellar habitat.
Colonization arks using replication
technology could organize the entire Asteroid Belt (and many
other bodies as well) into ark mass in less than 104 years [24],
hence the Belt and especially its larger members such as Ceres
or Pallas should be missing, yet this is not observed. Most
small-scale environmental effects would be either short-lived or
else virtually indistinguishable from natural processes, and in
any case low-volume and low-mass, hence extremely difficult to
detect.
The sole exception is derelict
machinery indisputably of alien manufacture. However,
interstellar arks are necessarily highly closed efficient
systems and would recycle rather than discard old machinery. A
replicative interstellar probe factory will minimize creation of
mechanical refuse to mini-raise production time, and will also
recycle for reasons of efficiency.
Exploitative and colonizing
replicative systems are ruled out observationally and hence
cannot leave derelict machinery. Mishaps producing derelicts,
artificial debris or other tangible evidence are improbable when
a mature technology is employed. An intriguing alternative is
biological markers.
Extraterrestrial data concealed in
DNA, representing a low-mass self-replicating artifact, has been
suggested as a carrier of extraterrestrial information [25, 28],
but attempts to decode possible “virus messages” have been
unsuccessful [29].
Further, such messages are unstable
even over short periods of time due to thermal degradation or
spontaneous cross-linkage; only the genetic code itself, which
could contain at most a very simple message (perhaps 100 bits),
has remained stable over geological times.
2. 3 Passive Artifacts
The simplest class of passive artifact is monuments, including
simple inert blocks or sculptures like the “extraterrestrial
message block” displayed at the National Air and Space Museum in
Washington, D.C. [30], corner reflectors which reflect optical
beams back towards the transmitter no matter from what direction
they originate [31], or isotope missives in which the isotopic
composition of material samples are encoded to yield information
upon being read by a mass spectrometer, up to 2000 bits per
alloy sample [28].
Other passive artifacts might
include buried alien databanks [32] or marker buoys and beacons
- to tag mineral deposits, waste dumps, equipment caches, or to
transmit navigational or warning signals [22]. Another possible
class is the highly redundant passive artifact.
This might consist of a large number
of relatively simple (presumably physically small) objects whose
purpose is to announce the existence of their creators but may
also include a brief communication encoded as isotopic missives
or genetic messages. If a band of artificial tektites were found
on the Moon or the Earth it might be possible to deduce the
existence of ETI, the age of the artifacts, and their
direction of travel [33].
Large numbers compensate for hostile
environments, and might be an inexpensive way to leave a marker
behind. Passive artifacts are unlikely to be observed in the
Solar System. If their purpose is merely to leave a calling
card, a solar-powered radio or visible light beacon in Earth
orbit (or some other equally glaringly obvious marker) would be
least ambiguous and most likely to be detected.
This alternative can be ruled out on
observational grounds. If the purpose is to transmit some body
of information to the finders [76], then a purely inactive
device with no secure means of avoiding dangerous environmental
exigencies and no self-repair capabilities would be unlikely to
survive long enough to complete its designated mission.
Finally, sophisticated
extraterrestrial engineers, having reached out across
interstellar distances to investigate the Solar System, are
unlikely to leave behind only a passive artifact incapable of
providing constant surveillance of an interesting, “valuable”
inhabited star system.
2. 4 Active Probes
A probe is a physical device which observes and reports back to
its senders. Another possible function is interaction with the
subjects under observation, either further to test and observe
them or to influence their development in some way. Such
influences may be benign [34] or otherwise [35].
The requirements for monitoring an
entire star system, and of reporting back to the sending
civilization, rule out purely biological probes, though these
could possibly be deployed as subprobes [36] under the control
of a mechanical system. To survive, probes must be active and
self-repairing but need not be self-reproducing, although the
capacity of a machine to reproduce is inherent in the logic of
self-repair [24].
Active artifacts incapable of
self-repair are insufficiently durable and will not be sent. A
civilization able to conduct a programme of vigorous
interstellar exploration using vehicles which are expensive to
build and launch takes decades or centuries to reach their
destinations, and which must perform exceedingly complex tasks
upon arrival must be highly skilled in automata engineering.
NASA has investigated self-testing
and repairing (STAR) computers for deep-space missions [37], as
did the Daedalus design group in connection with a 100-year
interstellar mission [38]. A recent NASA Systems Feasibility
Study concluded that spacecraft self-repair,
self-reconfiguration, and even self-reproduction are feasible
technological goals by the year 2000 AD [24].
Lofgren [39] has shown that a
self-repairing, self-reproducing automaton system can possess a
theoretically unbounded operational lifespan. Technically
proficient ETI should be able to design very-long-lifespan
selfrepairing machine systems [5].
The discovery of accidental
environmental effects caused by the passage or presence of the probe
is improbable because such efforts are likely to have been foreseen
and avoided. “Spent” devices like Lunar Ranger [40] are ruled out by
the self-repair requirement. Similarly, impact devices are unlikely
because it seems pointless to send a probe light-years just to end
in destruction, although impacting deployed subprobes cannot be
ruled out.
Crewed probes [14, 19] are less
efficient than automata, thus are unlikely for large-scale
exploration. Repeater stations (perhaps part of a galactic
communications network), telemetry stations, and purely educational
databanks, intended primarily not to observe but to teach (upon some
given trigger signal or event) are not inconsistent with the notion
of an active self-repairing probe and most likely represent some of
its many functions. In any case, all have similar observational
consequences.
Active, self-repairing interstellar
probes are the most likely class of observable ETI artifact
in the Solar System. This result permits us to devise a specific
observational programme to experimentally validate the Artifact
Hypothesis.
3. WHERE
SHOULD WE LOOK?
A spherical Solar System boundary enclosing the orbit of Pluto
consists of 260,000 AU3 of mostly empty interplanetary space and
1011 km2 of planetary and asteroidal surface area. To test the
Artifact Hypothesis observationally, in theory this entire space
should be combed for extraterrestrial probes.
Fortunately most regions may be
logically excluded from the search because of an extremely low
probability of occupation, thus reducing the search for
extraterrestrial artifacts (SETA) to manageable proportions.
Clarke
[41] and Bracewell [42] suggest an equipartition of effort between
senders and recipients in which the sender is required only to send
probes through target star systems on hyperbolic orbits and
potential recipients are held responsible for detection, the
initiation of dialogue, and possibly capture.
However, even if the probe decelerates
in one year from 10%c to solar escape velocity upon approaching the
Solar System, the required reaction energy from a l-ton rocket
emitted as a point source of solar-spectrum radiation would appear
as a +24 magnitude object at 100 AU and a +19 magnitude object at 10
AU. Detection is improbable in the extreme.
The minimum reasonable velocity for
heliocentric hyperbolic orbits is that of intramercurial solar
escape velocity, about 0.1 AU/day. For a perfectly reflecting 10
square meter body at full phase angle, the threshold detection
radius for the GEODSS-prototype automated asteroid search system (mB
= +16.5) [43] is 0.01 AU.
The object will cross this volume, if at
all, in five hours or less with a mean probability of detection of 7
x 10-5. Even at the limiting magnitude of the Space Telescope the
threshold range is 2 AU, a detection sphere which the probe crosses
in no more than one month. If the probe does not decelerate from
greater than 10%c, visual detection must take place in minutes at
most, nearly hopeless even if the artifact employs a radio beacon as
an aid to acquisition.
The amount and quality of data obtained
for the builders of a flyby interstellar probe are quite limited
[44], and the idea that probes pass by only briefly on their way to
other stars makes little sense in view of the tremendous distances
which must be covered to reach the Solar System [45]. Flyby probes
thus must be regarded as unsuitable for missions of long-term
exploration and surveillance.
Following deceleration and initial system survey, an active probe
capable of self-repair will elect to reside in the best possible
location to monitor phenomena relevant to its mission to seek out
life and intelligent species. This location may include heliocentric
orbits, phanetocentric orbits, or surface sites.
In keeping with the Principle of Economy
[5] the artifact must represent the simplest possible mechanism
necessary to perform the mission and will act to maximize the
probability of success through longevity and hazard avoidance.
Hence the search space of a SETA effort
to detect extraterrestrial artifacts must conform to two criteria
[11] which have well-defined observational consequences:
(1) Ability to consistently
monitor environments most likely to harbor or to evolve
intelligent life
(2) Maximum artifact lifespan with minimum complexity
Present indications are that the only
Solar System site where life has existed for aeons is the Earth,
although simple life may exist elsewhere, perhaps on Mars, Titan, or
Jupiter, possibly employing some exotic biochemistry [46-48].
These other planets are very
interesting, but Earth is clearly the most exotic and complex so the
terrestrial environment must be regarded as the principal target for
continuous surveillance by the probe. In view of the technical and
scientific competence of its designers the probe must be presumed to
have correctly recognized the significance of our planet and to have
taken up residence nearby.
-
Criterion (1) thus requires the artifact
to be sited either in orbit near Earth or the Moon, or in an orbit
which frequently carries it close enough to Earth to permit adequate
periodic surveillance. Terrestrial surface sites are unlikely
because these would restrict the ability of the probe to
continuously monitor the entire environment.
(Even if the main probe
were not situated near Earth, it would likely deploy permanent
surveillance subprobes in our vicinity which would then be
detectable by the less demanding search programme proposed here).
-
Criterion (2) requiring maximum lifespan
implies that the artifact will attempt to spend as much time as
possible in regions of low environmental hazard - e.g., minimum
high-energy particle... intensities and electric and magnetic field
densities, and minimum danger from micrometeorite and debris
impacts. Titis rules out the siting of artifacts in planetary
magnetospheres or ring systems.
Also, to max-greige lifespan the
artifact must have access to sufficient energy. Self-contained
systems are unlikely to provide enough power for data processing,
self-repair operations, orbit/attitude control and interstellar
radio transmission. An onboard fusion power plant is possible, but
most likely the artifact will collect solar energy, hence must
reside near the Sun.
This requirement, as well as criterion
(1), eliminates all outer planet sites. Similarly, orbits with
intramercurial aphelia may be dynamically stable and yet must be
rejected - Poynting-Robertson drag alone could remove such bodies as
large as 100 meters over geological timescales [49]. The artifact
should operate with maximum efficiency, so long-term (> 105 yr),
stable parking orbits are preferred to orbits which demand the
continuous expenditure of propulsive energy for station-keeping.
This eliminates most heliocentric orbits.
Further, a self-repairing probe need only be thermodynamically open
to energy - in principle, rising structural or material entropy can
be countered by a sufficient application of low-entropy solar
energy. New mass is required only to replace negligible losses due
to impact spallation, degassing or volatilization, and accidental or
purposeful ejection, so access to large stores of matter as on
planetary surfaces or near small asteroids or comets is unnecessary.
Minimum organizational and operational complexity demands that the
artifact not site itself in locations which may require it to
undertake major external construction projects as a general product
factory [24]. In principle, a replication-class artifact could
install itself on a planetary surface with the intention of building
its own shielding, communications gear, subprobes [44], transport
and propulsion mechanisms, using a general product factory
industrial complex constructed locally by itself.
However, from a hardware standpoint this
method of operation is less preferable since it introduces
additional failure modes into the mission plan, requires the
construction of a factory, and imposes more severe resource
requirements.
Criterion (2) also argues strongly
against sitting the artifact on the surface of any celestial body
having
(a) an appreciable escape velocity requiring a major
propulsion system for deorbit or ascent
(b) an appreciable
atmosphere requiring complex additional maintenance systems for
continuous protection from degradative chemical, biological,
thermal, erosional, hydrological, climatic and geological events, or
rotation, clouds, and electromagnetic phenomena which may inhibit
continuous access to solar energy and which may interfere with the
artifact’s ability to observe or to transmit progress reports.
Several factors are less significant in
fixing the SETA search volume. For instance, cosmic ray intensity is
roughly constant throughout the Solar System, except within
planetary magnetospheres. The solar wind velocity changes
imperceptibly between 1-5 AU, with ion temperature falling by a
factor of two and mean ion density decreasing according to the
inverse square law [50].
The meteorite flux varies only 1-3
orders over the same heliocentric range [5], less within orbital
regions of major interest. Finally, probable orbital insertion
trajectories for incoming probes are unimportant because propulsion
systems capable of interstellar flight are presumed capable of minor
orbital plane corrections such as ecliptic alignment after or during
final deceleration.
The potential search volume for extraterrestrial artifacts thus
reduces to five distinct orbital classes [11]:
(1) Geocentric orbits located
between two Earth-centered concentric spheres of radii
70,000 km and 326,400 km
(2) selenocentric orbits between 3000-58,100 km lunar
altitude
(3) stable synodic libration orbits around Earth-Moon
Lagrangian points L4 and L5
(4) Earth-Moon halo orbits near collinear Lagrangian points
LI and L2
(5) Sun-Earth L4/L5 Lagrangian orbits
This is where we should begin our search
for alien artifacts.
In addition, there are several low-probability categories of
planet-crossing and other orbits which could possibly prove suitable
as long-term parking orbits for extraterrestrial automata. Wetherill
[52] has shown that the Earth-approaching Aten, Apollo and Amor
asteroids have orbital lifetimes on the order of 107-108 years, and
number > 105 in sizes > 100 meters.
One special orbit of interest is the
unusual resonance of asteroid 1685 Toro with Earth (8:5) and Venus
(13:5) which appears to be stabilised by close approaches to within
9 x l05 km of Earth twice every eight years [53, 54]. The orbit has
been found stable for integration times up to 5000 years, and it is
believed that Mars perturbations set an upper limit of 3 x 105 years
for the librations [55].
Other Earth-approaching librating
asteroids such as 887 Alinda have also been studied [56]. Searches
for objects in stable orbits between Earth and Venus have been
proposed [57], and a circular orbit at 0.85 AU has been suggested
for the long-term storage of nuclear wastes [58-59], although the
claimed I OS-year stability is suspect on the basis of previous
numerical experiments [60].
Recent investigation of the problem of
satellites of asteroids’ (possible 104-107 year stability) [61-62]
also could have potential relevance to SETI searches of intramartian
orbits. However, objects in Earth-approaching heliocentric orbits
spend too much time too far from both Sun and Earth, should have
shorter lifetimes than bodies in geocentric, selenocentric, or
Lagrangian orbits, and hence are unlikely to have survived long
enough to be observable by us.
4. STATUS OF THE
SEARCH
The formal observational status of the first four of the five
primary orbital sites for extraterrestrial probes is given in Ref.
11 and will not be reviewed here, except to note that the record is
woefully incomplete. Preliminary recent SETA searches of three of
the potential sites [10, 63] have given negative results to date,
but this work is far from complete.
Future direct optical searches may begin by filling the enormous
gaps in the observational record using ground-based instruments (cf.
[64] ). However, detection of the smallest likely probe in
geocentric, selenocentric, and Earth/ Moon Lagrangian orbits implies
a search to magnitude +27 to +28, which requires the Space Telescope
[65] or equivalent technology.
Selenocentric probes could more easily
be detected using a lunar-based (surface or orbiting) telescope
facility because proximity to the target reduces the required
magnitude limits to +17 to +23 for an exhaustive search [11]. The
proposed 300-inch Very Large Space Telescope (VLST) [66] would only
permit the certain detection of 10-20 meter, low-reflectivity
artifacts parked in Sun-Earth Lagrangian orbits, so exhaustive
ground-based or Earth-orbit-based searches are not feasible.
However, a large space telescope with
limiting magnitude +29 stationed at Sun/ Earth L4/L5 could guarantee
an exhaustive search for small artifacts over a time period of about
a century. Radar and infrared observations [11] offer few
significant improvements over visual searches.
A few past proposals have emphasized
observing probe emissions rather than the probe itself. Bracewell
[67] suggested that the well-known long-delay echo (LDE) phenomenon
was of the type which might be expected as a call sign from an
extraterrestrial artifact parked in Earth orbit and desiring to
communicate, and Lunan [68] claimed to have decoded several “LDE
messages” based on data from Stormer [69] and van de Pol [70].
Lawton and Newton [71] performed a
series of LDE experiments and concluded the reflection signals were
of a purely physical nature, though they later proposed [72] that
radio call signals should be transmitted to likely probe positions
in an attempt to stimulate a response.
Kardashev reported receiving “coded
signals” from within the Solar System and of possibly alien origin
[73], but Western experts believed the signals came from secret U.S.
military communications satellites or from magnetospheric energy.
discharge [74].
Kuiper and Morris [12] proposed
intercepting radio communications between alien probes in the Solar
System and their extrasolar senders, but admitted the alien signals
may be spread so widely in frequency that they would be very
difficult to detect with a modest antenna.
Targeted radio listening searches could also be conducted of likely
probe residence orbits in an eavesdropping mode to detect accidental
electromagnetic leakage radiation. Searches for radio beacons could
establish indirect limits on the existence of probes in the Solar
System - the all-sky survey outlined in [75] would provide
observational limits on the minimum size of a solar-powered
Earth-Moon orbiting artifact maintaining its own local acquisition
beacon.
5. SUMMARY AND
CONCLUSIONS
The Artifact Hypothesis states that a technologically advanced
extraterrestrial civilization has undertaken a long-term programme
of galactic exploration via transmission of material artifacts. Four
general classes of unconcealed observable artifacts are potentially
available to test this Hypothesis: Astroengineering activities,
self-replicating artifacts, passive artifacts, and active probes.
Of these, only active self-repairing
probes are likely both to exist and to be observable from within the
Solar System. Fortunately the search for alien exploratory probes
does not require combing the entire Solar System.
Rationally-designed artifacts will elect to deploy themselves where
they can consistently monitor those environments most likely to harbour or to evolve intelligent life, and where they can anticipate
maximum lifespan with minimum complexity.
Flyby probes are improbable, so the
potential search volume for extraterrestrial artifacts reduces to
five orbital classes including geocentric, selenocentric, Earth-Moon
L4/L5 libration, Earth-Moon LI/L2 halo, and Sun-Earth L4/L5 orbits.
The present observational status of each of these orbital classes is
inadequate. Preliminary searches have begun but this work is far
from complete. Future direct optical searches should fill the
enormous gaps in the observational record using ground-based
instruments, space telescopes, lunar-based telescopes, and direct
probes to hunt for artifacts, and other indirect means to eavesdrop
or intercept alien radio communications originating from within the
Solar System.
ACKNOWLEDGEMENTS
The author thanks Ronald N. Bracewell, Robert S. Harrington, Michael
D. Papagiannis, Robert G. Roosen, Clyde W. Tombaugh, Francisco
Valdes, and George W. Wetherill for their comments on an earlier
version of this manuscript.
REFERENCES
1. Cocconi, G. and Morrison, P.,
Searching for interstellar communications, Nature, 184, 844-846
(1959).
2. Oliver, B. M. and Billingham, J. (Eds.), Project Cyclops; A
Design Study of a System for Detecting Extraterrestrial
Intelligent
Life, revised edition, NASA CR-114445, 1973.
3. Morrison, P., Billingham, J. and Woffe, J. (Eds.), The Search
for Extraterrestrial Intelligence, SETI, NASA SP419, 1977.
4. Billingham, J. (Ed.), Life in the Universe, MIT Press,
Cambridge, Massachusetts, 1981.
5. Freitas, R. A. Jr., The case for interstellar probes, JBIS,
36, 490=495 (1983).
6. Hart, M. H., An explanation for the absence of
extraterrestrials on Earth, Quart. if. Roy. Astr. Sot., 16,
128-135 (1975).
7. Tiplet, F. J., Extraterrestrial intelligent beings do not
exist, Quart. if. Roy. Astr. Soc., 21,267-281 (1980).
8. Hart, M. H. and Zuckerman, B. (Eds.), Extraterrestrial: Where
Are They? Pergamon Preu, New York, 1982.
9. Freitas, R. A. Jr., Extraterrestrial intelligence in the
Solar System: Resolving the Fermi Paradox,,IBlS, 36, 496-500
(1983).
10. Freitas, R. A. Jr. and Valdes, F., A search for natural or
artificial objects located at the Earth-Moon librartion points,
Icarus,
42, 442447 (1980).
11. Freitas, R. A. Jr., If they are here, where are they?
Observational and search considerations, Icarus, 55, in press,
1983.
12. Kuiper, T. B. H. and Morris, M., Searching for
extraterrestrial civilizations, Science, 196, 616-621 (1977).
13. R.A. Freitas, Jr., Terraforming Mars and Venus using machine
serf-replicating systems (SRS),.IBIS, 36, 139-142 (1983).
14. Stephenson, D. G., Extraterrestrial cultures within the
Solar System?, Quart, J. Roy. Asst. Soc., 20, 422428 (1979).
15. Papagiannis, M.D., Are we all alone, or could they be in the
Asteroid Belt?, Quart. J. Roy. Astr. Soc., 19, 277-281 (1978).
16. Stephenson, D. G., Models of interstellar exploration,
Quart. J. Roy. Astr. Soc., 23, 236-251 (1982).
17. Tiplet, F. J., Extraterrestrial intelligence: The debate
continues, Phys Today, 35, 3, 34-38 (1982).
18. McCall, R. and Asimov, I., Our World in Space, New York
Graphic Society, Ltd., Greenwich, Connecticut, 1974.
19. Matloff, G. I.., Utilization of O'Neill's Model I Lagrange
point colony as an interstellar ark, JBIS, 29, 775-785 (1976).
20. De San, M. G., Hypothesis on the Origin of UFOs, Editecs,
Bologna, Italy, 1979.
21. De San, M. G., The ultimate destiny of an intelligent
species - everlasting nomadic life in the Galaxy, JBIS, 34,
219-237
(1981).
22. Foster, G. V., Non-human artifacts in the Solar System,
Spaceflight, 14, 447453 (1972).
23. Freitas, R. A. Jr., A serf-reproducing interstellar probe,
JBIS, 33, 251-264 (1980).
24. Freitas, R. A. Jr. and Gilbreath, Wm. P. (Eds.), Advanced
Automation for Space Missions: Final Report, NASA CP-2255,
1982.
25. Edie, L. C, Messages from other worlds, Science, 136, 184
(1962).
26. Kross, R. D, Space messengers, Science, 136,913-914 (1962).
27. Crick, F. H. C, and Orgei, L. E., Directed panspermia,
Icarus, 19, 341-346 (1973).
28. Marx, G., Message through time, Acta Astronautics, 6, 221-225
(1979).
29. Yokoo, H. and Oshima, T., Is bacteriophage phi-X174 DNA a
message from an extraterrestrial intelligence?, Icarus, 38, 148-
153 (1979).
30. Dooling, D, Speculating on Man's neighhours, Spaceflight,
17, 231-232, 240 (1975).
31. Anderson, C. W., A relic interstellar corner reflector in
the Solar System?, Mercury, 3, SewOct, 2-3 (1974).
32. Saunders, M. W., Databank for an inhabited extrasolar
planet; Purpose, indication, and installation, JBIS, 30, 349-358
(1977).
33. Bracewell, R. N., Personal communication, 1982.
34. Clarke, A. C., 2001: A Space Odyssey, New American Library,
New York, 1968.
35. Benford, G., In the Ocean of Night, Dell, New York, 1977.
36. Clarke, A. C., Rendezvous with Rams, Ballantine, New York,
1973.
37. Avizienis, A, Gilley, G. C., Mathur, F. P., Rennels, D. A.,
Rohr, J. A. and Rubin, D. K., The STAR (Self-Testing-And-
Repairing) computer: An investigation of the theory and practice
of fault-tolerant computer design, IEEE Trans, Comp., C-
20,1312-1321 (1971).
38. Grant, T. J., Project Daedalus: The computers, In A. R.
Martin (Ed.), Project Daedalus - The final report on the BIS
starship
study, JBlS, Interstellar Studies Supplement, London, England,
S130-S142, 1978.
39. Lofgren, L., Kinematic and tessellation models of
serf-repair, in E. E. Bernard and M. R. Kate (Eds.),
Biologics! Prototypes
and Synthetic Systems, Volume 1, Plenum Press, New York,
342-369, 1962.
40. Roosen, R. G., Personal communication, 1982.
41. Clarke, A. C., An optimum strategy for interstellar robot
probes, JBIS, 31,438 (1978).
42. Bracewell, R. N., Manifestations of advanced civilizations,
in
J. Billingham (Ed.), Life tn the Universe, MIT Press, Cambridge,
Massachusetts, 343-350, 1981.
43. Taff, L G., A new asteroid observation and search technique,
Publ. Astron. Soc. Pac., 93, 658-660 (1981).
44. Martin, A. R. (Ed.), Project Daedalus - The final report on
the BIS starship study, JBIS, Interstellar Studies Supplement,
London, England, 1978.
45. Papagiannis, M.D., Could we be the only advanced
technological civilization in our galaxy?, in H. Noda (Ed.),
Origin of Life,
Proceedings of the 5th International Conference on the Origin of
Life, April, 1977, Kyoto, Japan, Center for Academic
Publications, Tokyo, 583-595, 1978.
46. Ponnameruma, C. and Molton, P., The prospect of life on
Jupiter, Ortgtns of Life, 4, 32-44 (1973).
47. Sagan, C. and Lederberg, J., The prospects for life on Mars:
A pre-Viking assessment, Icarus, 28, 291-300 (1976).
48. Feinberg, G. and Shapiro, R., Life Beyond Earth, William
Morrow, New York, 1980.
49. Weidenschilling, S..I., Iron/silicate fracfionation and the
origin of Mercury, Icarus, 35, 99-111 (1978).
50. Van Allen, J. A., Interplanetary particles and fields, Sci.
A mer. 233, 3, 160-173 (1975).
51. Dohnanyi, J.S., Interplanetary objects in review: Statistics
of their masses and dynamies, lcarus, 17, 1-48 (1972).
52. Wetherill, G. W., Steady state. populations of Apollo-Amor
objects, Icarus, 37, 96-112 (1979).
53.
Ip, W. H. and Mehra, R., Resonances and libration of some Apollo
and Amor asteroids with the Earth, Astron. J.,78, 142-147
(1973).
54. Danielson, L., The orbital resonances between the asteroid
Toro and the Earth and Venus, The Moon and the Planets, 18, 265-272 (1978).
55. Williams, J.G. and Wetherill, G. W., Physical studies of the
minor planets, XIII. Long-term orbital evolution of 1685 Toro,
Astron. J., 78, 510-515 (1973).
56. Janiczek, P.M., Seidelmann, P. K. and Duncombe, R. L.,
Resonances and encounters in the inner Solar System, Assrote J.,
77,
764-773 (1972).
57. Shoemaker, E. M. and Helin, E. F., Earth-approaching
asteroids as targets for exploration, in D. Morrison and C.
Wells (Eds.),
A steroids: A n Exploration Assessment, NASA Conference PubI.,
2053, 245-256, 1978.
58. Burns, R. E., Causey, W. E., Galloway, W. E. and Nelson, R.
W., Nuclear Waste Disposal tn Space, NASA Technical Paper
1225, 1978.
59. Priest, C. C., Nixon, R. F. and Rice, E. E., Space disposal
of nuclear wastes, Astronautics and Aeronautics, 18, 26-35,
(1980).
60. Birn, J., On the stability of the planetary system, Astron.
Astrophys, 24, 283-293 (1973).
61. Donnison, J.R., The satellite of Herculina, Mon. NoL Roy.
Astr. Soc., 186, 35P-37P (1979).
62. Van Flandern, T. C., Tedesco, E. F. and Binzel, R. P,
Satellites of asteroids, in T. Gehrels (Ed.), A steroids,
University of
Arizona Press, Tucson, 443-465, 1979.
63. Valdez, F. and Freitas, R. A. Jr., A search for objects near
the Earth-Moon Lagrangian points, lcarus, 53, 453-457 (1983).
64. Bagby, J.P., Natural Earth satellites, JBIS, 34, 289-293
(1981).
65. Bahcall, J.N. and Spitzer, L. Jr., The Space Telescope, Sci
Am., 247, 40-51 (1982).
66. Dooling, D., Giant orbiting telescopes considered for 1990s,
Star and Sky, 2, 8, 8-14 (1980).
67. Bracewell, R. N., Communications from superior galactic
communities, Nature, 186, 670-671 (1960).
68. Lunan, D., Man and the Start, Souvenir Press, London, 1974.
69. Stormer, C., Short wave echoes and the Aurora Borealis,
Nature, 122, 681 (1928).
70. 'Van der Pol, B., Short wave echoes and the Aurora Borealis,
Nature, 122, 878-879 (1928).
71. Lawson, A. T. and Newton, S. J, Long delayed echoes: The
search for a solution, Spaceflight, 16, 181-187 (1974).
72. Lawson, A. T. and Newton, S. J, Long delayed echoes - the
trojan ionosphere, JBIS, 27, 907-920 (1974).
73. A galactic sputnik? Spaceflight, 16, 105 (1974).
74. Belitsky, B., CETI in the Soviet Union, Spaceflight, 19,
193,196 (1977).
75. Wolfe, J. H., Edelson, R. E., Billingham, J., Crow, R. B.,
Gulkis, S., Olsen, E. T., Oliver, B. M., Peterson, A.M., Seeger,
C. L.
and Tarter, J. C., SETI - The search for extraterrestrial
intelligence: Plans and rationale. in J. Billingham (Ed.), Life
in the
Universe, MIT Press, Cambridge, Massachusetts, 391-415, 1981.
76. Sagan, C. (Ed.), Murmurs of Earth: The Voyager Interstellar
Record, Ballantine, New York, 1978.
|