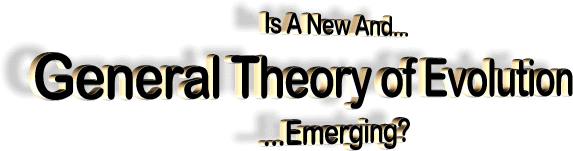
by Stephen Jay Gould
1980
from
HCCSouthwestLearningWeb Website
Abstract
The modern synthesis, as an exclusive
proposition, has broken down on both of its fundamental claims:
extrapolationism (gradual allelic substitution as a model for all
evolutionary change) and nearly exclusive reliance on selection
leading to adaptation.
Evolution is a hierarchical process with
complementary, but different, modes of change at its three major
levels: variation within populations, speciation, and patterns of
macroevolution. Speciation is not always an extension of gradual,
adaptive allelic substitution to greater effect, but may represent,
as Goldschmidt argued, a different style of genetic change - rapid
reorganization of the genome, perhaps non-adaptive.
Macroevolutionary trends do not arise from the gradual, adaptive
transformation of populations, but usually from a higher-order
selection operating upon groups of species, while the individual
species themselves generally do not change following their
geologically instantaneous origin. I refer to these two
discontinuities in the evolutionary hierarchy as the Goldschmidt
break (between change in populations and speciation) and the Wright
break (between speciation and trends as differential success among
species).
A new and general evolutionary theory will embody this notion of
hierarchy and stress a variety of themes either ignored or
explicitly rejected by the modern synthesis: punctuational change at
all levels, important non-adaptive change at all levels, control of
evolution not only by selection, but equally by constraints of
history, development and architecture - thus restoring to
evolutionary theory a concept of organism.
Stephen Jay Gould
Museum of Comparative Zoology
Harvard University, Cambridge,
Massachusetts 02138
Accepted: October 15, 1979
I - The Modern
Synthesis
In one of the last skeptical books written before the Darwinian tide
of the modern synthesis asserted its hegemony, Robson and Richards
characterized the expanding orthodoxy that they deplored:
The theory of Natural Selection...
postulates that the evolutionary process is unitary, and that
not only are groups formed by the multiplication of single
variants having survival value, but also that such divergences
are amplified to produce adaptations (both specializations and
organization). It has been customary to admit that certain
ancillary processes are operative (isolation, correlation), but
the importance of these, as active principles, is subordinate to
selection.
(1936, pp. 370-371).
Darwinism, as a set of ideas, is
sufficiently broad and variously denned to include a multitude of
truths and sins.
Darwin himself disavowed many
interpretations made in his name (1880, for example). The version
known as the "modern synthesis" or "Neo-Darwinism" (different from
what the late 19th century called Neo-Darwinism - see Romanes, 1900)
is, I think, fairly characterized in its essentials by Robson and
Richards.
Its foundation rests upon two major premises:
-
Point mutations
(micro-mutations) are the ultimate source of variability.
Evolutionary change is a process of gradual allelic
substitution within a population. Events at broader scale,
from the origin of new species to long-ranging evolutionary
trends, represent the same process, extended in time and
effect - large numbers of allelic substitutions
incorporated sequentially over long periods of time. In
short, gradualism, continuity and evolutionary change by the
transformation of populations.
-
Genetic variation is raw
material only. Natural selection directs evolutionary
change. Rates and directions of change are controlled by
selection with little constraint exerted by raw material
(slow rates are due to weak selection, not insufficient
variation). All genetic change is adaptive (though some
phenotypic effects, due to
pleiotropy, etc., may not be).
In short, selection leading to
adaptation.
All these statements, as Robson and Richards also note, are subject
to recognized exceptions - and this imposes a great
frustration upon anyone who would characterize the modern synthesis
in order to criticize it.
All the synthesists recognized
exceptions and "ancillary processes," but they attempted both to
prescribe a low relative frequency for them and to limit their
application to domains of little evolutionary importance. Thus,
genetic drift certainly occurs - but only in populations so
small and so near the brink that their rapid extinction will almost
certainly ensue.
And phenotypes include many non-adaptive
features by
allometry and pleiotropy, but all are epiphenomena of
primarily adaptive genetic changes and none can have any marked
effect upon the organism (for, if inadaptive, they will lead to
negative selection and elimination and, if adaptive, will enter the
model in their own right).
Thus, a synthesist could always deny a
charge of rigidity by invoking these official exceptions, even
though their circumscription, both in frequency and effect, actually
guaranteed the hegemony of the two cardinal principles.
This frustrating situation had been
noted by critics of an earlier Darwinian orthodoxy, by Romanes
writing of Wallace, for example (1900, p. 21):
[For Wallace,] the law of utility
is, to all intents and purposes, universal, with the result that
natural selection is virtually the only cause of organic
evolution. I say 'to all intents and purposes,' or 'virtually,'
because Mr. Wallace does not expressly maintain the abstract
impossibility of laws and causes other than those of utility and
natural selection; indeed, at the end of his treatise, he quotes
with approval Darwin's judgment, that 'natural selection has
been the most important, but not the exclusive means of
modification.' Nevertheless, as he nowhere recognizes any other
law or cause of adaptive evolution, he practically concludes
that, on inductive or empirical grounds, there is no such other
law or cause to be entertained.
Lest anyone think that Robson and
Richards, as doubters, had characterized the opposition unfairly, or
that their two principles represent too simplistic or unsubtle a
view of the synthetic theory, I cite the characterization of one of
the architects of the theory himself (Mayr 1963, p. 586 - the first
statement of his chapter on species and trans-specific evolution):
The proponents of the synthetic
theory maintain that all evolution is due to the accumulation of
small genetic changes, guided by natural selection, and that
trans-specific evolution is nothing but an extrapolation and
magnification of the events that take place within populations
and species.
The early classics of the modern
synthesis - particularly Dobzhansky's first edition (1937) and
Simpson's first book (1944) - were quite expansive, generous and
pluralistic.
But the synthesis hardened throughout the late 40's and
50's, and later editions of the same classics (Dobzhansky 1951;
Simpson 1953) are more rigid in their insistence upon micromutation,
gradual transformation and adaptation guided by selection (see Gould
1980 for an analysis of changes between Simpson's two books).
When
Watson and Crick then determined the structure of DNA, and when the
triplet code was cracked a few years later, everything seemed to
fall even further into place. Chromosomes are long strings of
triplets coding, in sequence, for the proteins that build organisms.
Most point mutations are simple base substitutions. A physics and
chemistry had been added, and it squared well with the prevailing
orthodoxy.
I well remember how the synthetic theory beguiled me with its
unifying power when I was a graduate student in the mid-1960's.
Since then I have been watching it slowly unravel as a universal
description of evolution. The molecular assault came first, followed
quickly by renewed attention to unorthodox theories of speciation
and by challenges at the level of macroevolution itself.
I have been
reluctant to admit it - since beguiling is often forever - but if Mayr's characterization of the synthetic theory is accurate, then
that theory, as a general proposition, is effectively dead, despite
its persistence as textbook orthodoxy.
II - Reduction and
Hierarchy
The modern synthetic theory embodies a strong faith in reductionism.
It advocates a smooth extrapolation across all levels and scales -
from the base substitution to the origin of higher
taxa.
The most
sophisticated of leading introductory textbooks in biology still
proclaims:
[Can] more extensive evolutionary
change, macroevolution, be explained as an outcome of these
microevolutionary shifts. Did birds really arise from reptiles
by an accumulation of gene substitutions of the kind illustrated
by the raspberry eye-color gene.
The answer is that it is entirely plausible, and no one has come
up with a better explanation... The fossil record suggests that
macroevolution is indeed gradual, paced at a rate that leads to
the conclusion that it is based upon hundreds or thousands of
gene substitutions no different in kind from the ones examined
in our case histories.
(Wilson et al. 1973, pp. 793-794)
The general alternative to such
reductionism is a concept of hierarchy - a world constructed not as
a smooth and seamless continuum, permitting simple extrapolation
from the lowest level to the highest, but as a series of ascending
levels, each bound to the one below it in some ways and independent
in others.
Discontinuities and seams characterize the transitions;
"emergent" features not implicit in the operation of processes at
lower levels, may control events at higher levels. The basic
processes - mutation, selection, etc. - may enter into explanations
at all scales (and in that sense we may still hope for a general
theory of evolution), but they work in different ways on the
characteristic material of divers levels (see Bateson 1978 and
Koestler 1978, for all its other inadequacies, for good discussions
of hierarchy and its anti-reductionistic implications; Eldredge and
Cracraft 1980).
The molecular level, which once seemed through its central dogma and
triplet code to provide an excellent "atomic" basis for smooth
extrapolation, now demands hierarchical interpretation itself.
The
triplet code is only machine language (I thank E. Yates for this
appropriate metaphor).
The program resides at a higher level of
control and regulation - and we know virtually nothing about it.
With its inserted sequences and jumping genes, the genome contains
sets of scissors and pots of glue to snip and unite bits and pieces
from various sources.
Thirty to seventy percent of the mammalian
genome consists of repetitive sequences, some repeated hundreds or
thousands of times. What are they for (if anything)? What role do
they play in the regulation of development? Molecular biologists are
groping to understand this higher control upon primary products of
the triplet code. In that understanding, we will probably obtain a
basis for styles of evolutionary change radically different from the
sequential allelic substitutions, each of minute effect, that the
modern synthesis so strongly advocated.
The uncovering of hierarchy
on the molecular level will probably exclude smooth continuity
across other levels. (We may find, for example, that structural gene
substitutions control most small-scale, adaptive variation within
local populations, while disruption of regulation lies behind most
key innovations in macro-evolution.)
The modern synthesis drew most of its direct conclusions from
studies of local populations and their immediate adaptations.
It
then extrapolated the postulated mechanism of these adaptations -
gradual, allelic substitution - to encompass all larger-scale
events. The synthesis is now breaking down on both sides of this
argument. Many evolutionists now doubt exclusive control by
selection upon genetic change within local populations.
Moreover,
even if local populations alter as the synthesis maintains, we now
doubt that the same style of change controls events at the two major
higher levels: speciation and patterns of macroevolution.
III - A Note
on Local Populations and Neutrality
At the level of populations, the synthesis has broken on the issue
of amounts of genetic variation.
Selection, though it eliminates
variation in both its classical modes (directional and, especially,
stabilizing) can also act to preserve variation through such
phenomena as overdominance, frequency dependence, and response to
small-scale fluctuation of spatial and temporal environments.
Nonetheless, the copiousness of genetic variation, as revealed first
in the electrophoretic techniques that resolve only some of it (Lewontin
and Hubby 1966; Lewontin 1974), cannot be encompassed by our models
of selective control (of course, the models, rather than nature, may
be wrong). This fact has forced many evolutionists, once stout
synthesists themselves, to embrace the idea that alleles often drift
to high frequency or fixation, and that many common variants are
therefore neutral or just slightly deleterious.
This admission lends
support to a previous interpretation of the approximately even
ticking of the molecular clock (Wilson 1977) - that it reflects the
neutral status of most changes in structural genes rather than a
grand averaging of various types of selection over time.
None of this evidence, of course, negates the role of conventional
selection and adaptation in molding parts of the phenotype with
obvious importance for survival and reproduction. Still, it rather
damps Mayr's enthusiastic claim for "all evolution... guided by
natural selection."
The question, as with so many issues in the
complex sciences of natural history, becomes one of relative
frequency.
Are the Darwinian substitutions merely a surface skin on
a sea of variation invisible to selection, or are the neutral
substitutions merely a thin bottom layer underlying a Darwinian
ocean above? Or where in between?
In short, the specter of stochasticity has intruded upon
explanations of evolutionary change.
This represents a fundamental
challenge to Darwinism, which holds, as its very basis, that random
factors enter only in the production of raw material, and that the
deterministic process of selection produces change and direction
(see Nei 1975).
IV - The Level of
Speciation and the Goldschmidt Break
Ever since
Darwin called his
book The Origin of Species, evolutionists have regarded the
formation of reproductively isolated units by speciation as a
fundamental process of large-scale change.
Yet speciation occurs at
too high a level to be observed directly in nature or produced by
experiment in most cases.
Therefore, theories of speciation have
been based on analogy, extrapolation and inference. Darwin himself
focused on artificial selection and geographic variation. He
regarded subspecies as incipient species and viewed their gradual,
accumulating divergence as the primary mode of origin for new taxa.
The modern synthesis continued this tradition of extrapolation from
local populations and used the accepted model for adaptive
geographic variation - gradual allelic substitution directed by
natural selection - as a paradigm for the origin of species.
Mayr's
(1942, 1963) model of allopatric speciation did challenge Darwin's
implied notion of sympatric continuity. It emphasized the crucial
role of isolation from gene flow and did promote the importance of
small founding populations and relatively rapid rates of change.
Thus, the small peripheral isolate, rather than the large local
population in persistent contact with other conspecifics, became the
incipient species.
Nonetheless, despite this welcome departure from
the purest form of Darwinian gradualism, the allopatric theory held
firmly to the two major principles that permit smooth extrapolation
from the
Biston betularia model of adaptive, allelic substitution:
-
The accumulating changes that lead to speciation are adaptive.
Reproductive isolation is a consequence of sufficient accumulation
-
Although aided by founder effects and even (possibly) by drift,
although dependent upon isolation from gene flow, although
proceeding more rapidly than local differentiation within large
populations, successful speciation is still a cumulative and
sequential process powered by selection through large numbers of
generations.
It is, if you will, Darwinism a little faster.
I have no doubt that many species originate in this way; but it now
appears that many, perhaps most, do not. The new models stand at
variance with the synthetic proposition that speciation is an
extension of microevolution within local populations.
Some of the
new models call upon genetic variation of a different kind, and they
regard reproductive isolation as potentially primary and
non-adaptive rather than secondary and adaptive. Insofar as these
new models be valid in theory and numerically important in
application, speciation is not a simple "conversion" to larger
effect of processes occurring at the lower level of adaptive
modeling within local populations. It represents a discontinuity in
our hierarchy of explanations, as the much maligned Richard
Goldschmidt argued explicitly in 1940.
There are many ways to synthesize the swirling set of apparently
disparate challenges that have rocked the allopatric orthodoxy and
established an alternative set of models for speciation.
The
following reconstruction is neither historically sequential nor the
only logical pathway of linkage, but it does summarize the
challenges - on population structure, place of origin, genetic
style, rate, and relation to adaptation - in some reasonable order.
-
Under the allopatric
orthodoxy, species are viewed as integrated units which, if
not actually panmictic, are at least sufficiently
homogenized by gene flow to be treated as entities.
This
belief in effective homogenization within central
populations underlies the
allopatric theory with its
emphasis on peripheral isolation as a precondition for
speciation. But many evolutionists now believe that gene
flow is often too weak to overcome selection and other
intrinsic processes within local demes (Ehrlich and Raven
1969).
Thus, the model of a large, homogenized central
population preventing local differentiation and requiring allopatric "flight" of isolated demes for speciation may not
be generally valid. Perhaps most local demes have the
required independence for potential speciation.
-
The primary terms of reference
for theories of speciation - allopatry and sympatry -
lose their meaning if we accept the first statement.
Objections to sympatric speciation centered upon the
homogenizing force of gene flow.
But if demes may be
independent in all geographic domains of a species, then sympatry loses its meaning and allopatry its necessity.
Independent demes within the central range (sympatric by
location) function, in their freedom from gene flow, like
the peripheral isolates of allopatric theory.
In other
words, the terms make no sense outside a theory of
population structure that contrasts central panmixia with
marginal isolation. They should be abandoned.
-
In this context "sympatric"
speciation loses its status as an extremely improbable
event. If demes are largely independent, new species may
originate anywhere within the geographic range of an
ancestral form.
Moreover, many evolutionists now doubt that parapatric distributions (far more common then previously
thought) must represent cases of secondary contact.
White
(1978, p. 342) believes that many, if not most, are primary
and that speciation can also occur between populations
continually in contact if gene flow can be overcome either
by strong selection or by the sheer rapidity of potential
fixation for major chromosomal variants (see White, 1978, p.
17 on clinal speciation).
-
Most "sympatric" models of
speciation are based upon rates and styles of genetic change
inconsistent with the reliance placed by the modern
synthesis on slow, or at least sequential change.
The most exciting entry among
punctuational models for speciation in ecological time is
the emphasis, now coming from several quarters, on
chromosomal alterations as isolating mechanisms (White 1978;
Bush 1975; Carson 1975, 1978; Wilson et al. 1975; Bush et
al. 1977) - sometimes called the theory of chromosomal
speciation.
In certain population structures, particularly
in very small and circumscribed groups with high degrees of
inbreeding, major chromosomal changes can rise to fixation
in less than a handful of generations (mating of
heterozygous F1 sibs to produce F2 homozygotes for a start).
Allan Wilson, Guy Bush and their colleagues (Wilson et al.
1975; Bush et al. 1977) find a strong correlation between
rates of karyotypic and anatomical change, but no relation
between amounts of substitution in structural genes and any
conventional assessment of phenotypic modification, either
in speed or extent.
They suggest that speciation may be more
a matter of gene regulation and rearrangement than of
changes in structural genes that adapt local populations in
minor ways to fluctuating environments (the Biston betularia
model).
Carson (1975, 1978) has also stressed the importance of
small demes, chromosomal change, and extremely rapid
speciation in his founder-flush theory with its emphasis on
extreme bottlenecking during crashes of the flush-crash
cycle (see Powell 1978 for experimental support).
Explicitly contrasting this view
with extrapolationist models based on sequential
substitution of structural genes, he writes (1975, p. 88):
-
Most theories of speciation
are wedded to gradualism, using the mode of origin of intraspecific adaptations as a model...
I would
nevertheless like to propose... that speciational events
may be set in motion and important genetic saltations
towards species formation accomplished by a series of
catastrophic, stochastic genetic events... initiated
when an unusual forced reorganization of the epistatic
supergenes of the closed variability system occurs...
I
propose that this cycle of disorganization and
reorganization be viewed as the essence of the
speciation process.
-
Another consequence of such
essentially
saltational origin is even more disturbing to
conventional views than the rapidity of the process itself,
as Carson has forcefully stated. The control of evolution by
selection leading to adaptation lies at the heart of the
modern synthesis.
Thus, reproductive isolation,
the definition of speciation, is attained as a by-product of
adaptation - that is, a population diverges by sequential
adaptation and eventually becomes sufficiently different
from its ancestor to foreclose interbreeding. (Selection for
reproductive isolation may also be direct when two
imperfectly-separate forms come into contact.)
But in saltational, chromosomal
speciation, reproductive isolation comes first and cannot be
considered as an adaptation at all. It is a stochastic event
that establishes a species by the technical definition of
reproductive isolation. To be sure, the later success of
this species in competition may depend upon its subsequent
acquisition of adaptations; but the origin itself may be
non-adaptive.
We can, in fact, reverse the conventional view
and argue that speciation, by forming new entities
stochastically, provides raw material for selection.
These challenges can be summarized in the claim that a
discontinuity in explanation exists between allelic
substitutions in local populations (sequential, slow and
adaptive) and the origin of new species (often discontinuous
and non-adaptive). During the heyday of the modern
synthesis, Richard Goldschmidt was castigated for his
defense of punctuational speciation.
I was told as a
graduate student that this great geneticist had gone astray
because he had been a lab man with no feel for nature, a
person who hadn't studied the adaptation of local
populations and couldn't appreciate its potential power, by
extrapolation, to form new species.
But I discovered, in
writing
Ontogeny and Phytogeny, that Goldschmidt had spent a
good part of his career studying geographic variation,
largely in the coloration of lepidopteran larvae (where he
developed the concept of rate genes to explain minor changes
in pattern).
I then turned to his major book (1940) and
found that his defense of saltational speciation is not
based on ignorance of geographic variation, but on an
explicit study of it; half the book is devoted to this
subject. Goldschmidt concludes that geographic variation is
ubiquitous, adaptive, and essential for the persistence of
established species.
But it is simply not the stuff of
speciation; it is a different process. Speciation,
Goldschmidt argues, occurs at different rates and uses
different kinds of genetic variation. We do not now accept
all his arguments about the nature of variation, but his
explicit anti-extrapolationist statement is the epitome and
foundation of emerging views on speciation discussed in this
section.
There is a discontinuity in cause and explanation
between adaptation in local populations and speciation; they
represent two distinct, though interacting, levels of
evolution.
We might refer to this
discontinuity as the Goldschmidt break, for he wrote:
-
The characters of subspecies
are of a gradient type, the species limit is
characterized by a gap, an unbridged difference in many
characters. This gap cannot be bridged by theoretically
continuing the subspecific gradient or cline beyond its
actually existing limits. The subspecies do not merge
into the species either actually or ideally...
Micro-evolution by
accumulation of micromutations - we may also say
neo-Darwinian evolution - is a process which leads to
diversification strictly within the species, usually, if
not exclusively, for the sake of adaptation of the
species to specific conditions within the area which it
is able to occupy... Subspecies are actually,
therefore, neither incipient species nor models for the
origin of species.
They are more or less
diversified blind alleys within the species.
The decisive step in
evolution, the first step towards macroevolution, the
step from one species to another, requires another
evolutionary method than that of sheer accumulation of
micro-mutations.
(1940, p. 183).
V -
Macroevolution and the Wright Break
The extrapolationist model of macroevolution views trends and major
transitions as an extension of allelic substitution within
populations - the march of frequency distributions through
time.
Gradual change becomes the normal state of species.
The
discontinuities of the fossil record are all attributed to its
notorious imperfection; the remarkable stasis exhibited by most
species during millions of years is ignored (as no data), or
relegated to descriptive sections of taxonomic monographs.
But
gradualism is not the only important implication of the extrapolationist model.
Two additional consequences have channeled
our concept of macroevolution, both rather rigidly and with
unfortunate effect.
-
First, the trends and transitions of
macroevolution are envisaged as events in the phyletic mode -
populations transforming themselves steadily through time. Splitting
and branching are acknowledged to be sure, lest life be terminated
by its prevalent extinctions.
But splitting becomes a device for the
generation of diversity upon designs attained through "progressive"
processes of transformation. Splitting, or cladogenesis, becomes
subordinate in importance to transformation, or
anagenesis (see
Ayala 1976, p. 141; but see also Mayr 1963, p. 621 for a rather
lonely voice in the defense of copious speciation as an input to "progressive"
evolution).
-
Secondly, the adaptationism that prevails in
interpreting change in local populations gains greater confidence in
extrapolation. For if allelic substitutions in ecological time have
an adaptive basis, then surely a unidirectional trend that persists
for millions of years within a single lineage cannot bear any other
interpretation.
This extrapolationist model of adaptive, phyletic gradualism has
been vigorously challenged by several paleobiologists - and again
with a claim for discontinuity in explanation at different levels.
The general challenge embodies three loosely united themes:
-
Evolutionary trends as a
higher level process: Eldredge and I have argued (1972, and
Gould and Eldredge 1977) that imperfections of the record
cannot explain all discontinuity (and certainly cannot
encompass stasis). We regard stasis and discontinuity as an
expression of how evolution works when translated into
geological time. Gradual change is not the normal state of a
species.
Large, successful central populations undergo minor
adaptive modifications of fluctuating effect through time
(Goldschmidt's "diversified blind alleys"), but they will
rarely transform in toto to something fundamentally new.
Speciation, the basis of macroevolution, is a process of
branching.
And this branching, under any current model of
speciation - conventional allopatry to chromosomal saltation
- is so rapid in geological translation (thousands of years
at most compared with millions for the duration of most
fossil species) that its results should generally lie on a
bedding plane, not through the thick sedimentary sequence of
a long hillslope.
(The expectation of gradualism emerges as
a kind of double illusion. It represents, first of all, an
incorrect translation of conventional
allopatry. Allopatric
speciation seems so slow and gradual in ecological time that
most paleontologists never recognized it as a challenge to
the style of gradualism - steady change over millions of
years - promulgated by custom as a model for the history of
life. But it now appears that "slow" allopatry itself may be
less important than a host of alternatives that yield new
species rapidly even in ecological time.)
Thus, our model of
"punctuated equilibria" holds that evolution is concentrated
in events of speciation and that successful speciation is an
infrequent event punctuating the stasis of large populations
that do not alter in fundamental ways during the millions of
years that they endure.
But if species originate in geological instants and then do
not alter in major ways, then evolutionary trends cannot
represent a simple extrapolation of allelic substitution
within a population. Trends must be the product of
differential success among species (Eldredge and Gould 1972;
Stanley 1975).
In other words, species themselves must be
inputs, and trends the result of their differential origin
and survival.
Speciation interposes itself as an irreducible
level between change in local populations and trends in
geological time. Macroevolution is, as Stanley argues (1975,
p. 648), decoupled from microevolution.
Sewall Wright recognized the hierarchical implications of
viewing species as irreducible inputs to macroevolution
when he argued (1967, p. 121) that the relationship between
change in local populations and evolutionary trends can only
be analogical. Just as mutation is random with respect to
the direction of change within a population, so too might
speciation be random with respect to the direction of a
macroevolutionary trend.
A higher form of selection, acting
directly upon species through differential rates of
extinction, may then be the analog of natural selection
working within populations through differential mortality of
individuals.
Evolutionary trends therefore represent a third level
superposed upon speciation and change within demes.
Intrademic events cannot encompass speciation because rates,
genetic styles, and relation to adaptation differ for the
two processes. Likewise, since trends "use" species as their
raw material, they represent a process at a higher level
than speciation itself.
They reflect a sorting out of
speciation events. With apologies for the pun, the
hierarchical rupture between speciation and macroevolutionary trends might be called the Wright break.
As a final point about the extrapolation of methods for the
study of events within populations, the cladogenetic basis
of macroevolution virtually precludes any direct
application of the primary apparatus for microevolutionary
theory: classical population genetics.
I believe that
essentially all macroevolution is
cladogenesis and its
concatenated effects. What we call "anagenesis," and often
attempt to delineate as a separate phyletic process leading
to "progress," is just accumulated cladogenesis filtered
through the directing force of species selection (Stanley
1975) - Wright's higher level analog of natural selection.
Carson (1978, p. 925) makes the point forcefully, again
recognizing Sewall Wright as its long and chief defender:
Investigation of cladistic events as opposed to phyletic (anagenetic)
ones requires a different perspective from that normally
assumed in classical population genetics.
The statistical
and mathematical comfort of the Hardy-Weinberg equilibrium
in large populations has to be abandoned in favor of the
vague realization that nearly everywhere in nature we are
faced with data suggesting the partial or indeed complete
sundering of gene pools. If we are to deal realistically
with cladogenesis we must seek to delineate each genetic and
environmental factor which may promote isolation.
The most
important devices are clearly those which operate at the
very lowest population level: sib from sib, family from
family, deme from deme. Formal population genetics just
cannot deal with such things, as Wright pointed out long
ago.
Eldredge (1979) has traced many conceptual errors and
prejudicial blockages to our tendency for conceiving of
evolution as the transformation of characters within
phyletic lineages, rather than as the origin of new taxa by
cladogenesis (the transformational versus the taxic view in
his terms).
I believe that, in ways deeper than we realize,
our preference for transformational thinking represents a
cultural tie to the controlling Western themes of progress
and ranking by intrinsic merit - an attitude that can be
traced in evolutionary thought to Lamarck's distinction
between the march up life's ladder promoted by the pouvoir
de la vie and the tangential departures imposed by influence des circonstances, with the first process
essential and the second deflective.
Nonetheless,
macro-evolution is fundamentally about the origin of
taxa by
splitting.
-
The
saltational initiation of
major transitions: The absence of fossil evidence for
intermediary stages between major transitions in organic
design, indeed our inability, even in our imagination, to
construct functional intermediates in many cases, has been a
persistent and nagging problem for gradualistic accounts of
evolution.
St. George Mivart (1871), Darwin's most cogent
critic, referred to it as the dilemma of "the incipient
stages of useful structures" - of what possible benefit to a
reptile is two percent of a wing?
The dilemma has two
potential solutions.
The first, preferred by Darwinians
because it preserves both gradualism and adaptation, is the
principle of pre-adaptation: the intermediary stages
functioned in another way but were, by good fortune in
retrospect, pre-adapted to a new role they could play only
after greater elaboration.
Thus, if feathers first
functioned "for" insulation and later "for" the trapping of
insect prey (Ostrom 1979), a proto-wing might be built
without any reference to flight.
I do not doubt the supreme importance of preadaptation, but
the other alternative, treated with caution, reluctance,
disdain or even fear by the modern synthesis, now deserves a
rehearing in the light of renewed interest in development:
perhaps, in many cases, the intermediates never existed.
I
do not refer to the saltational origin of entire new
designs, complete in all their complex and integrated
features - a fantasy that would be truly anti-Darwinian in
denying any creativity to selection and relegating it to the
role of eliminating old models. Instead, I envisage a
potential saltational origin for the essential features of
key adaptations.
Why may we not imagine that gill arch bones
of an ancestral
agnathan moved forward in one step to
surround the mouth and form proto-jaws? Such a change would
scarcely establish the Bauplan of the
gnathostomes.
So much
more must be altered in the reconstruction of agnathan
design - the building of a true shoulder girdle with bony,
paired appendages, to say the least. But the discontinuous
origin of a proto-jaw might set up new regimes of
development and selection that would quickly lead to other,
coordinated modifications.
Yet Darwin, conflating gradualism
with natural selection as he did so often, wrongly
proclaimed that any such discontinuity, even for organs
(much less taxa) would destroy his theory:
-
If it could be demonstrated that any complex organ existed,
which could not possibly have been formed by numerous,
successive, slight modifications, my theory would absolutely
break down (1859, p. 189).
During the past 30 years, such proposals have generally been
treated as a fantasy signifying surrender - an invocation of
hopeful monsters rather than a square facing of a difficult
issue.
But our renewed interest in development, the only
discipline of biology that might unify molecular and
evolutionary approaches into a coherent science, suggests
that such ideas are neither fantastic, utterly contrary to
genetic principles, nor untestable.
Goldschmidt conflated two proposals as causes for hopeful
monsters - "systemic mutations" involving the entire genome
(a spin-off from his fallacious belief that the entire genome
acted as an integrated unit), and small mutations with large
impact upon adult phenotypes because they work upon early
stages of ontogeny and lead to cascading effects throughout
embryology.
We reject his first proposal, but the second,
eminently plausible, theme might unite a Darwinian
insistence upon continuity of genetic change with a macroevolutionary suspicion of
phenetic discontinuity.
It
is, after all, a major focus in the study of
heterochrony
(effects, often profound, of small changes in developmental
rate upon adult phenotypes); it is also implied in the
emphasis now being placed upon regulatory genes in the
genesis of macroevolutionary change (King and Wilson 1975) -
for regulation is fundamentally about timing in the complex
orchestration of development.
Moreover, although we cannot
readily build "hopeful monsters," the subject of major
change through alteration of developmental rate can be
treated, perhaps more than analogically, both by experiment
and comparative biology.
The study of spontaneous
anomalies of development (teratology) and experimental
perturbations of embryogenic rates explores the tendencies and boundaries
of developmental systems and allows us to specify potential
pathways of macroevolutionary change (see, for example, the
stunning experiment of Hampe 1959, on recreation of
reptilian patterns in birds, after 200 million years of
their phenotypic absence, by experimental manipulations that
amount to alterations in rate of development for the
fibula).
At the very least, these approaches work with real
information and seem so much more fruitful than the
construction of adaptive stories or the invention of
hypothetical intermediates.
-
The importance of
non-adaptation: The emphasis on natural selection as the
only directing force of any importance in evolution led
inevitably to an analysis of all attributes of organisms as
adaptations.
Indeed, the tendency has infected our language,
for, without thinking about what it implies, we use
"adaptation" as our favored, descriptive term for
designating any recognizable bit of changed morphology in
evolution. I believe that this "adaptationist program" has
had decidedly unfortunate effects in biology (Gould and
Lewontin, 1979).
It has led to a reliance on speculative
storytelling in preference to the analysis of form and its
constraints; and, if wrong, in any case, it is virtually
impossible to dislodge because the failure of one story
leads to invention of another rather than abandonment of the
enterprise.
Yet, as I argued earlier, the hegemony of adaptation has
been broken at the two lower levels of our evolutionary
hierarchy: variation within populations, and speciation.
Most populations may contain too much variation for
selection to maintain; moreover, if the neutralists are even
part right, much allelic substitution occurs without
controlling influence from selection, and with no direct
relationship to adaptation.
If species often form as a
result of major chromosomal alterations, then their origin -
the establishment of reproductive isolation - may require no
reference to adaptation.
Similarly, at this third level of macroevolution, both arguments previously cited against the
conventional extrapolationist view require that we abandon
strict adaptationism.
-
If trends are produced by the unidirectional
transformation of populations (orthoselection), then they
can scarcely receive other than a conventional adaptive
explanation.
After all, if adaptation lies behind single
allelic substitutions in the
Biston betularia model for
change in local populations, what else but even stronger,
more persistent selection and adaptive orientation can
render a trend that persists for millions of years? But if
trends represent a higher-level process of differential
origin and mortality among species, then a suite of
potentially non-adaptive explanations must be considered.
Trends, for example, may occur because some kinds of species
tend to speciate more often than others. This tendency may
reside in the character of environments or in attributes of
behavior and population structure bearing no relationship to
morphologies that spread through lineages as a result of
higher speciation rates among some of their members. Or
trends may arise from the greater longevity of certain kinds
of species.
Again, this greater persistence may have little
to do with the morphologies that come to prevail as a
result.
I suspect that many morphological trends in
paleontology - a bugbear of the profession because we have
been unable to explain them in ordinary adaptive terms - are
non-adaptive sequelae of differential species success based
upon environments and population structures.
-
If transitions represent the continuous and gradual
transformation of populations, then they must be regulated
by adaptation throughout (even though adaptive orientation
may alter according to the principle of pre-adaptation).
But
if discontinuity arises through shifts in development, then
directions of potential change may be limited and strongly
constrained by the inherited program and developmental
mechanics of an organism.
Adaptation may determine whether
or not a hopeful monster survives, but primary constraint
upon its genesis and direction resides with inherited
ontogeny, not with selective modeling.
VI - Quo Vadis?
My crystal ball is clouded both by the dust of these growing
controversies and by the mists of ignorance emanating from molecular
biology, where even the basis of regulation in eukaryotes remains
shrouded in mystery.
I think I can see what is breaking down
in evolutionary theory - the strict construction of the modern
synthesis with its belief in pervasive adaptation, gradualism, and
extrapolation by smooth continuity from causes of change in local
populations to major trends and transitions in the history of life.
I do not know what will take its place as a unified theory, but I
would venture to predict some themes and outlines.
The new theory will be rooted in a
hierarchical view of nature. It will not embody the depressing
notion that levels are fundamentally distinct and necessarily
opposed to each other in their identification of causes (as the
older paleontologists held in maintaining that macro-evolution could
not, in principle, be referred to the same causes that regulate
microevolution - e.g., Osborn 1922).
It will possess a common body of causes
and constraints, but will recognize that they work in
characteristically different ways upon the material of different
levels - intrademic change, speciation, and patterns of
macroevolution.
As its second major departure from current orthodoxy, the new theory
will restore to biology a concept of organism. In an exceedingly
curious and unconscious bit of irony, strict selectionism (which
was not, please remember, Darwin's own view) debased what had been a
mainstay of biology - the organism as an integrated entity exerting
constraint over its history.
St. George Mivart expressed the subtle
point well in borrowing a metaphor from Galton. I shall call it Galton's polyhedron.
Mivart writes (1871, pp. 228-229):
This conception of such internal and
latent capabilities is somewhat like that of Mr. Galton...
according to which the organic world consists of entities, each
of which is, as it were, a spheroid with many facets on its
surface, upon one of which it reposes in stable equilibrium.
When by the accumulated action of incident forces this
equilibrium is disturbed, the spheroid is supposed to turn over
until it settles on an adjacent facet once more in stable
equilibrium. The internal tendency of an organism to certain
considerable and definite changes would correspond to the facets
on the surface of the spheroid.
Under strict selectionism, the organism
is a sphere. It exerts little constraint upon the character of its
potential change; it can roll along all paths.
Genetic variation is
copious, small in its increments, and available in all directions -
the essence of the term "xandom" as used to guarantee that
variation serves as raw material only and that selection controls
the direction of evolution.
By invoking Galton's polyhedron, I recommend no return to the
antiquated and anti-Darwinian view that mysterious "internal"
factors provide direction inherently, and that selection only
eliminates the unfit (orthogenesis, various forms of vitalism and
finalism). Instead, the facets are constraints exerted by the
developmental integration of organisms themselves.
Change cannot
occur in all directions, or with any increment; the organism is not
a metaphorical sphere. When the polyhedron tumbles, selection may
usually be the propelling force. But if adjacent facets are few in
number and wide in spacing, then we cannot identify selection as the
only, or even the primary control upon evolution.
For selection is
channeled by the form of the polyhedron it pushes, and these
constraints may exert a more powerful influence upon evolutionary
directions than the external push itself. This is the legitimate
sense of a much maligned claim that "internal factors" are important
in evolution. They channel and constrain Darwinian forces; they do
not stand in opposition to them.
Most of the other changes in
evolutionary viewpoint that I have advocated throughout this paper
fall out of Galton's metaphor: punctuational change at all levels
(the flip from facet to facet, since homeostatic systems change by
abrupt shifting to new equilibria); essential non-adaptation, even
in major parts of the phenotype (change in an integrated organism
often has effects that reverberate throughout the system);
channeling of direction by constraints of history and developmental
architecture.
Organisms are not billiard balls, struck in
deterministic fashion by the cue of natural selection, and rolling
to optimal positions on life's table. They influence their own
destiny in interesting, complex, and comprehensible ways.
We must
put this concept of organism back into evolutionary biology.
Literature
Cited
AYALA, F. J. 1976. Molecular
genetics and evolution. Pp. 1-20.
In: Ayala, F. J., ed. Molecular Evolution. Sinauer Associates;
Sunderland, Mass.
BATESON, G. 1978. Mind and Nature. E. P.
Dutton; New York. BUSH, G. L. 1975. Modes of animal speciation. Annu. Rev. Ecol.
Syst. 6:339-364.
BUSH, G. L., S. M. CASE, A. C. WILSON, AND J. L. PATTON.
1977. Rapid speciation and chromosomal evolution in mammals.
Proc. Nat. Acad. Sci. 74:3942-3946.
CARSON, H. L. 1975. The
genetics of speciation at the diploid level.
Am. Nat. 109:83-92.
CARSON, H. L. 1978. Chromosomes and species formation. Evolution.
32:925-927.
DARWIN, C. 1859. On the Origin of Species. 490 pp. John Murray;
London.
DARWIN, C. 1880. Sir Wyville Thomson and natural selection.
Nature 23:32.
DOBZHANSKY, TH. 1937. Genetics and the Origin of Species. 364
pp. Columbia Univ. Press; New York.
DOBZHANSKY, TH. 1951. Genetics and the Origin of Species. (3rd
ed.). 364 pp. Columbia Univ. Press; New York.
EHRLICH, P. R. AND P. H. RAVEN. 1969. Differentiation of
populations. Science. 165:1228-1232.
ELDREDGE, N. 1979. Alternative approaches to evolutionary theory.
Bull. Carnegie Mus. Nat. Hist. pp. 7-19.
ELDREDGE, N. AND J. CRACRAFT. 1980. Phylogenetic Patterns and
the Evolutionary Process. Columbia Univ. Press; New York.
ELDREDGE, N. AND S. J. GOULD. 1972. Punctuated equilibria: An
alternative to phyletic gradualism. Pp. 82-115. In: Schopf, T.
J. M., ed. Models in Paleobiology. Freeman, Cooper and Co.; San
Francisco, California.
GOLDSCHMIDT, R. 1940. The Material Basis of Evolution. 436 pp.
Yale Univ. Press; New Haven, Conn.
GOULD, S. J. 1980. G. G. Simpson, paleontology and the modern
synthesis. In: Mayr, E., ed. Conference on the making of the
Modern Synthesis. Harvard Univ. Press; Cambridge, Mass.
GOULD, S. J. AND N. ELDREDGE. 1977. Punctuated equilibria: the
tempo and mode of evolution reconsidered. Paleobiology.
3:115-151.
GOULD, S. J. AND R. C. LEWONTIN. 1979. The spandrels of San
Marco and the Panglossian paradigm: A critique of the
adapta-tionist program. Proc. R. Soc. London. 205:581-598.
HAMPE, A. 1959. Contribution a l'etude du developpement et de la
regulation des deficiences et des excedents dans la patte de
l'embryonlle poulet. Arch. Anat. Microsc. Morphol. Exp.
4&:345-478.
KING, M. C. AND A. C. WILSON. 1975. Evolution at two levels in
humans and chimpanzees. Science. 188:101-lib.
KOESTLER, A. 1978. Janus: a Summing Up. Random House; New York.
LEWONTIN, R. C. 1974. The Genetic Basis of Evolutionary Change.
346 pp. Columbia Univ. Press; New York.
LEWONTIN, R. C. AND J L. HUBBY. 1966. A molecular approach to
the study of genie heterozygosity in natural populations. II.
Amount of variation and degree of heterozygosity in natural
populations of Drosophila pseudoobscura. Genetics. 54:595-609.
MAYR, E. 1942. Systematics and the Origin of Species. 334 pp.
Columbia Univ Press; New York.
MAYR, E. 1963. Animal Species and Evolution. 797 pp. Belknap
Press of Harvard Univ. Press; Cambridge, Mass.
MIVART, ST. G. 1871. On the Genesis of Species. 296 pp.
MacMillan; London.
NEI, M. 1975. Molecular Population Genetics and Evolution.
American Elsevier; New York.
OSBORN, H. F. 1922. Orthogenesis as observed from
paleontolog-ical evidence beginning in the year 1889. Am. Nat.
56:134-143.
OSTROM, J H. 1979. Bird flight: How did it begin. Am. Sci.
67:46-56.
POWELL, J. R. 1978. The founder-flush speciation theory: an
experimental approach. Evolution. 32:465-474.
ROBSON, G. C. AND O. W. RICHARDS. 1936. The Variation of Animals
in Nature. Longmans, Green, and Co.; London.
ROMANES, G. J. 1900. Darwin and after Darwin, vol. 2.
Post-Darwinian questions. Heredity and Utility. 344 pp.
Longmans, Green, and Co.; London.
SIMPSON, G. G. 1944. Tempo and Mode in Evolution. 237 pp.
Columbia Univ. Press; New York.
SIMPSON, G. G. 1953. The Major Features of Evolution. 434 pp.
Columbia Univ. Press; New York.
STANLEY, S. M. 1975. A theory of evolution above the species
level. Proc. Nat. Acad. Sci. 72:646-650.
WHITE, M. J. D. 1978. Modes of Speciation. 455 pp. W.H. Freeman;
San Francisco, California.
WILSON, A. C, G. L. BUSH, S. M. CASE, AND M. C. KING. 1975.
Social structuring of mammalian populations and rate of
chromosomal evolution. Proc. Nat. Acad. Sci. 72:5061-5065.
WILSON, A. C, S. S. CARLSON, AND T. J. WHITE. 1977. Biochemical
evolution. Annu. Rev. Biochem. 46:573-639.
WILSON, E. O. ET AL. 1973. Life on Earth. Sinauer Associates;
Sunderland, Mass.
WRIGHT, S. 1967. Comments on the preliminary working papers of
Eden and Waddington. In: Moorehead, P. S. and M. M. Kaplan, eds.
Mathematical Challenges to the Neo-Darwinian Theory of
Evolution. Wistar Inst. Symp. 5:117-120.
|